15.3: Life Beyond Earth
( \newcommand{\kernel}{\mathrm{null}\,}\)

https:/pixabay.com/illustrations/alien-life-form-alien-planet-cosmos-3287912;
15.3.1 Life in Our Solar System
Are there any possible locations in our solar system outside of Earth where life may be found? Many scientists think that a few other worlds may be possible candidates to search for life. The four leading candidates are:
Mars: Today, Mars is a frozen desert with an atmosphere too thin to allow for liquid water to exist on the surface. Any liquid water released on the surface of Mars would quickly freeze or evaporate. However, our studies of Mars have found abundant evidence that Mars had a thicker atmosphere and warmer conditions and that liquid water flowed on the surface. Even though we have not found definitive evidence of life on Mars, it is possible that fossilized remains of microbes could be found on Mars. In addition, recent radar surveys have found evidence for liquid water beneath the surface. This water is likely heavily laden with salts that lower its freezing point, making it inhospitable to most life on Earth, but perhaps some extremophiles could still survive in these waters.
Europa: As noted in Chapter 12, tidal forces acting on Europa produce frictional heating and there is evidence of a liquid water beneath its icy crust. The tidal heating may not only melt some of its internal water, but it may also provide a source of energy to power life. These conditions might be conducive to extremophiles using a similar biochemistry as those found in volcanic sea vents in the deep oceans of Earth.
Enceladus: Like Europa, there is evidence of a liquid water ocean beneath the icy crust of Enceladus due to tidal heating. In addition, the Voyager probe found geysers of water erupting from the south pole of this moon of Saturn. So, while confirming the existence of life on Europa would require us to drilling through kilometers of ice, we could fly a probe close enough to take evidence of life in those geysers.
Titan: Saturn’s largest moon is very cold, covered with “rocks” made of ice and cryovolcanoes of cold “lava” flows on the surface. However, it also has a thick atmosphere and pools of liquid hydrocarbons on the surface. It may be that some cold-loving extremophile microbes could find a way to surface in that environment.
When people think of aliens, they usually think of something like little green men. However, if life exists on any of the other planets or moons in the Solar System, it is most likely to be single-celled organisms. Multicellular organisms are very unlikely, and we can now confidently rule out the possibility of any intelligent life in the Solar System.
To find an intelligent alien species with which we may one day communicate, we will have to look outside our Solar System to the exoplanets. The biggest difficulty to communicating with alien life though, is the distances. The closest star to the Sun is Proxima Centauri, which is about 4 light years away. Recently, a potentially Earth-sized planet has been discovered around Proxima Centauri. However, any message we might send to aliens on this world is limited by the speed of light. That means, if we sent a message today, it would not reach Proxima Centauri until four years later. Then, it would take another four years for their reply to reach us. An eight-year gap in sending messages make meaningful communication difficult and that is our best case scenario.
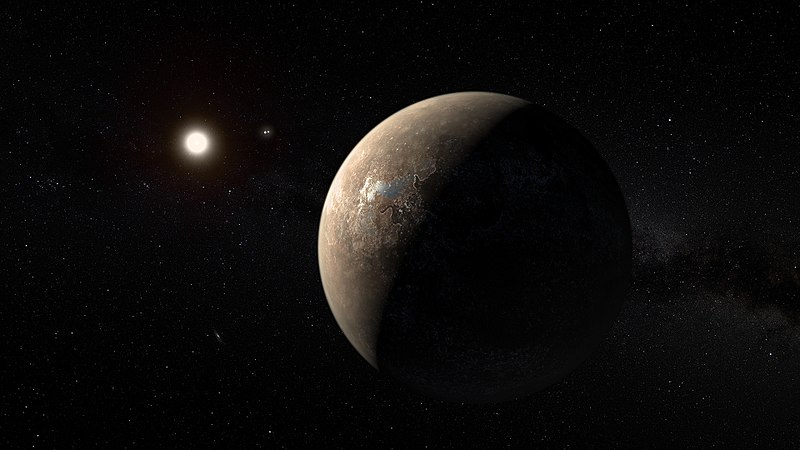
As of 1 August 2018, there are 3,815 confirmed exoplanets in 2,853 systems, with 633 systems having more than one planet. The first exoplanets were found around exotic bodies like pulsars and most of the early exoplanets were “hot Jupiters,” gas giants orbiting close to their stars. That was a limitation of our early detection methods and limited data. These planets were easy to detect because their gravity caused a large “wobble” in the star’s movement.
Using Kepler and other telescopes, scientists now look for exoplanets based on the slight dimming of a star’s brightness as the planet transits in front of it. This enables us to infer the planet’s size, orbital period, and density. Today, the most common exoplanet discovered is a “Super Earth,” a planet between Earth and Neptune in size. As we gather and analyze more data, we expect to find more Earth-sized planets throughout the galaxy.
There are billions of stars in the galaxy and it now appears that most stars have planetary systems. Odds are good that there should be many planets in the “goldilocks zones” capable of supporting life. Therefore, the galaxy should be teeming with life.
But where should we look for it?
What are the best candidate stellar systems?
And what are the odds that there exists an alien civilization with which we can communicate?
15.3.2 Drake Equation
.jpg?revision=1&size=bestfit&width=794&height=446)
https:/commons.wikimedia.org/wiki/File:Europa_Rising_-_Drake_Equation_(14486519161).jpg;
The first attempt to estimate the probability of intelligent life in our galaxy was formulated by Frank Drake. The Drake equation is a series of estimates of factors that must be present for a long-lasting technological civilization to arise. Multiplying them together would give us an estimate of on the number of intelligent civilizations in the galaxy. These seven factors are:
- R* = The rate of star formation.
- fp = Fraction of stars having planetary systems.
- ne = Number of habitable planets per planetary system.
- fl = Fraction of habitable planets where life emerges.
- fi = Fraction of inhabited planets where intelligence emerges.
- fc = Fraction of intelligent civilizations that develops and uses technology.
- L = The average lifespan of a technological civilization.
Since Drake originally proposed this equation in the 1960s, we have gained considerable information for the first couple of factors, but we still have almost no information about the remaining factors.
For example, we can estimate the rate of star formation by dividing population of Milky Way by its present age, giving us an average rate of star formation of about 10 new stars per year.
For the fraction of stars having planetary systems, we have detected thousands of planets around other stars. We have not surveyed the entire galaxy, but with the data collected so far and our current model for star formation lead us to expect that planets are a necessary by product of star formation. As a result, we expect most star systems to have formed planets as well. Therefore, we will assume that all stars have planets around them and assign this factor a value near 1.
The number of habitable planets per planetary system is a bit more difficult. Stars come in numerous sizes. The smallest detected star, SCR 1845–6357 A, has a mass of 0.084 times that of the Sun. The most massive star, R136a1, has a mass of 315 times that of the Sun. Smaller stars dimmer and emit less light, and as a result, have a too-small habitable zone, the orbital region around a star where temperatures can exist to allow for life as we know it. They also tend to poor in “metals,” what astronomers call elements heavier than helium, which would be necessary for life. In addition, larger stars a too-short lifetime. While our Sun has an overall expected lifetime of about 10-15 billion years, the massive blue-white stars have lifespans of only a few hundred million years. Since the evidence indicates that life took around a billion years to first appear on Earth, these stars would be too short-lived for life to emerge on any of their planets. This would limit us to stars close to the Sun in mass, which are those classified as A-, F-, G-, and K-type stars (Our Sun is a G2 type star).
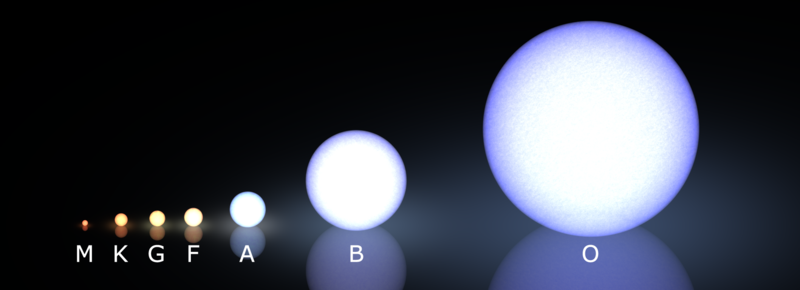

In addition, there are galactic habitable zones. Too close the galactic core and there is too much radiation. Too far away from the center and there will be too few of the heavier elements necessary for life.
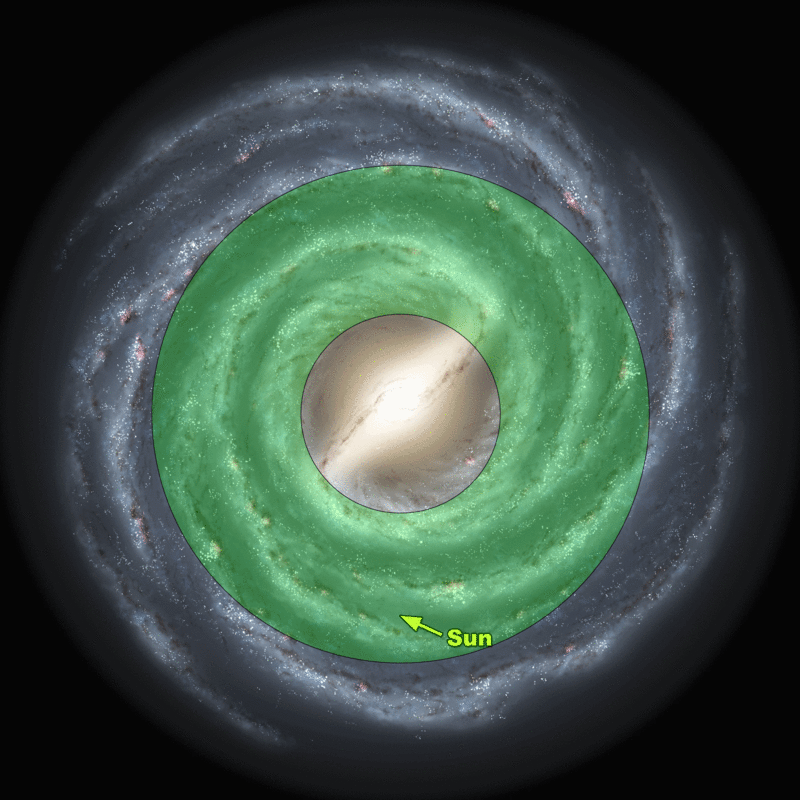
Finally, we could probably eliminate binary star systems. It is very unlikely that a planet in a binary system would have a stable orbit unless it is extremely close to one star (an S-Type orbit), or very far away from both (a P-Type orbit). Either orbit would take it outside of the Goldilocks zone.

Planets orbiting a binary star system can orbit both stars at once (P-Type) or tightly around just one star (S-Type)
As a result, we will give this factor a value of 1/10: one habitable planet in every 10 planetary systems.
We know nothing about the fraction of habitable planets on which life arises. Experiments like the Miller-Urey experiment suggest that this may be quite likely. However, we only have a sample size of one. We do not know if life is likely to emerge any where conditions are right for it to emerge. The fact that life appears able to get a toehold wherever it can on Earth and has endured through five mass extinction events, indicates that life may be common in the galaxy. As a result, we will continue to be optimistic and give this factor a value of 1.
For the fraction of life-bearing planets where intelligence arises, we have no facts, just speculation and opinion. There is no indication that intelligence is a necessary result of evolution and life on Earth existed for billions of years before our species appeared a mere 200,000 to 300,000 years ago. However, we will continue being optimistic, assume that life inevitably evolves wherever life appears, and assign this factor a value of 1.
Again, we have no facts about the next factor: the fraction of planets where intelligent life develops and uses technology. Also, we must consider that even though our species has been around for thousands of years, we only developed the technology to communicate over long distances around a century ago. Image an alien species sending us a message using radio waves that arrived here five hundred years ago, during the time of Galileo, no one would have heard it. However, it does seem reasonable to assume that intelligent life will develop technology sooner or later. So, we will give this factor a value of 1 also.
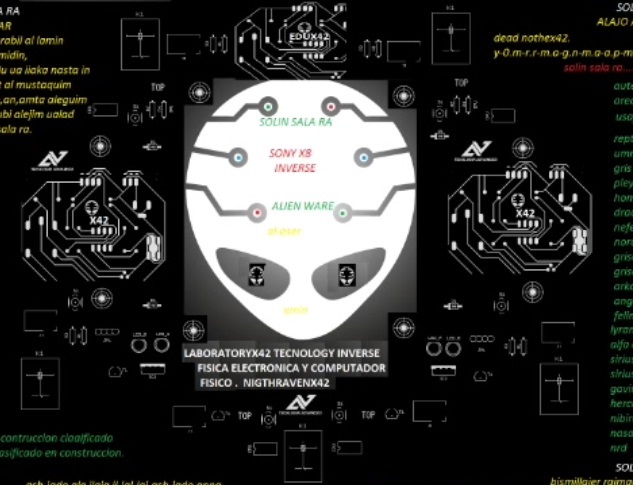
We don't know how many intelligent, technological civilizations exist in our galaxy.
https:/commons.wikimedia.org/wiki/File:Tecnologia_inversa.jpg;
Multiplying the values we have assigned to the first six factors gives us a result of:
10 X 1 X 1/10 X 1 X 1 X1 = 1
Therefore, using these optimistic assumptions, we can estimate that the number of intelligent, technological civilizations in the galaxy = the average lifespan of such a civilization. For this final factor, we cannot even use ourselves as an example: Our civilization has been technological for about 100 years, but who knows how long it will last? We could run out of energy or cause or civilization to collapse through war, global warming, or a plague within this century. Or we could discover solutions to these problems and have a technological civilization that lasts for thousands of years.
If the average lifetime of a technological civilization is 1 million years, there should be a million such civilizations in our galaxy. This would give us a galaxy teeming with civilizations! However, given the size of the galaxy, these civilizations would be spaced about 30 pc, or 100 light years, apart on average. This means that any two-way communication will take about 200 years (if there is in fact a technological civilization 100 light-years or less away from us). So, even with the most optimistic assumptions, the possibility of finding an alien civilization with which we may be able to communicate is very low.
Also, we assigned optimistic values to several very uncertain factors; even if only one of them is low, the number of expected civilizations drops quickly.