13.4: Nuclear Fusion
- Page ID
- 31139
13.4.1 The Four Fundamental Forces in Nature
The energy of the Sun comes from the fusion of hydrogen into helium. Nuclear fusion requires that like-charged nuclei get close enough to each other to fuse. Under normal conditions, the electromagnetic repulsion between two protons keeps them from coming close enough to stick together. Overcoming this repulsion requires extremely high temperatures of over 10 million kelvins.
Fusion in the sun involves the interaction of all four fundamental forces of nature.
The first force is gravity. While gravity dominates on the scale of planets and stars, in fact it is the weakest of the four forces. The reasons for its dominance on the large scale are that its always attractive (there is no “anti-gravity” force that has ever been found), its range is unlimited, and it increases with mass. Thus, the more massive objects are, the stronger the attraction due to gravity. The pressure from the gravitational collapse that formed the Sun generated the original heat that produced temperatures where fusion was possible. Today, the gravitation pressure of the Sun’s mass is in balance from the electromagnetic forces released by fusion in the core.
Electromagnetism is the other force we can recognize on the large scale. Unlike gravity, electromagnetism can either be attractive or repulsive. For example, opposite electric charges attract each other while opposite changes repel. Fluctuating electromagnetic fields produce all the forms of radiation from radio waves to visible light to high energy X-rays. Like gravity, the range of electromagnetism is unlimited, falling off with the inverse square of distance.
The final two forces have very short ranges and only operate at distances inside the radius of an atomic nuclear. As a result, they are called strong nuclear force and the weak nuclear force, respectively. The strong force holds tiny particles called quarks together to form the protons and neutrons in the nucleus. The weak force governs certain forms of radioactive decay, such as beta decay.
Each of the forces has a carrier particle associated with it. Electromagnetism has the photon while the strong nuclear force has the gluon (because they act as “glue” to hold the quarks together). The weak force is carried by W+, W-, and Z particles. The particle associated with gravity has been called the graviton, however, it has yet to be observed in nature.

13.4.2 Proton-Proton Chain
The process that powers most stars is a three-step fusion process called the proton–proton chain. The result of this chain is the fusing hydrogen nuclei (protons) in a single helium nucleus. The first step in the proton-proton chain involves two protons coming together to form a particle called a deuteron. As the two protons come together, the strong force causes them to stick while the weak force converts one of them into a neutron. To balance the charges, the deuteron emits a positron, the antimatter form of an electron that carries a positive charge. Fusing the two protons into a deuteron emits energy while the positron eventually encounters an election. When the matter and antimatter particles come together, they destroy each other, converting all their mass into a gamma photon. Another particle is also emitted from the formation of a deuteron, the neutrino. More about them later.
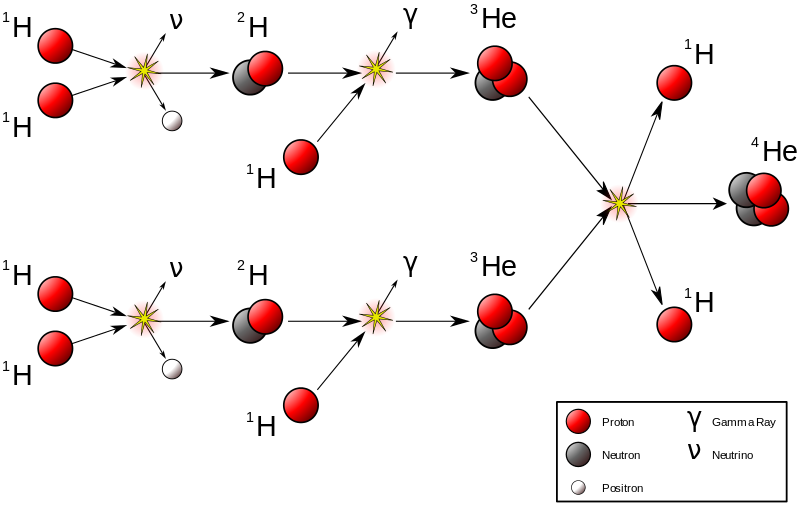
The second step involves the deuteron fusing with another proton, forming a helium-3 nucleus that contains two protons and one neutron. This also releases a burst of energy. Finally, two helium-3 nuclei will come together to form a helium-4 nucleus while ejecting two protons and releasing yet another burst of energy. The two free protons may fuse with other protons to continue the cycle.
In summary, the proton-proton chain requires a total input of four protons and produces an output of one helium-4 nucleus, 2 gamma rays, 2 positrons, and 2 neutrinos. The total mass of the output particles is 0.7% lower than the mass of the original four protons. This missing mass is converted into the energy that is emitted by the Sun.
Neutrinos are small, weakly interacting particles that are emitted directly from the core of the Sun and escape. Because they do not interact strongly interact with matter, neutrinos do not take a meandering path out of the core the way photons do. Instead, they travel straight out into space. Being able to observe these neutrinos gives us a direct picture of what is happening in the core. However, because they interact with matter so weakly, neutrinos are almost impossible to detect. Millions of neutrinos pass through the entire Earth every second without interacting with a single atom. They are no more likely to interact with Earth-based detectors than they are with the Sun. the only way to spot them is to have a huge detector volume and to be able to observe single interaction events. Neutrino detectors often employ 1,000 tonnes of water or other fluid deep underground where sensors look for a single flash of radiation indicating a neutrino detection.

Early searches for solar neutrinos, however, failed to find the predicted number. This created a problem for scientists, who either had to revise their models for the Sun’s interior or explain why their detectors were not finding the correct amount. Eventually, scientists realized that some of the neutrinos were changing form en route from the Sun and their detectors where only looking for the kind of neutrinos produced from the fusion itself. More recent observations find the right number of neutrinos by searching for different types of neutrinos.

https:/www.flickr.com/photos/berkeleylab/2826495494;