8.2: Three Modest Experiments
- Page ID
- 57744
elastic glass balls; inelastic wads off gum; weighing heat
A collision does not have to be violent to qualify for attention nor be exotic to make momenergy scorekeeping interesting. It is fun to begin with momenergy scorekeeping for three encounters of everyday kinds before strolling out onto the laboratory floor of high-energy particle physics.
Elastic collision: Momenergy automatically conserved
First Experiment: Elastic Collision. Suspend two identical glass marbles from automatically conserved the ceiling by two threads of the same length so that the marbles hang, at rest, just barely touching. Draw one back with the finger and release it (Figure 8-1). The released marble gathers speed. The speed peaks just as the first marble collides with the second. The collision is elastic: Total kinetic energy before the collision equals total kinetic energy after the collision. The elastic collision brings the first marble to a complete stop. The impact imparts to the second all the momentum the first one had. Conservation of momentum could not be clearer:
\[\left(\begin{array}{c} \text { total momentum } \\ \text { to the right just } \\ \text { before the collision, } \\ \text { all of it resident on } \\ \text { the first marble } \end{array}\right)=\left(\begin{array}{c} \text { total momentum } \\ \text { to the right just } \\ \text { after the collision, } \\ \text { all of it resident on } \\ \text { the second marble } \end{array}\right) \nonumber \]
And energy? In the collision the two particles exchange roles. The first particle comes to a halt. The second particle moves exactly as the first one did before the collision. Hence energy too is clearly conserved.
Just before the collision and just after: How do conditions compare? Same total momentum. Same total energy. Therefore same total momenergy.
Inelastic collision: Momenergy also conserved
Second Experiment: Inelastic Collision. Replace the two glass marbles by two also conserved identical balls of putty, wax, or chewing gum (Figure 8-2). Pull them aside by equal amounts and release.
Both released balls of chewing gum gather speed, moving toward one another. The equal and opposite velocities peak just before they collide with each other. By symmetry, the momentum of the right-moving particle has the same magnitude as the momentum of the left-moving particle. However, these momenta point in opposite directions. Regarded as vectors, they sum to zero. The momentum of the system therefore equals zero just before the collision.
Just after the collision? The two balls have stuck together. They are both at rest; each has zero momentum. Their combined momentum is also zero. In other words, the momentum of the system is zero after the collision. Zero it was also before the collision. Thus the momentum of the system is conserved.

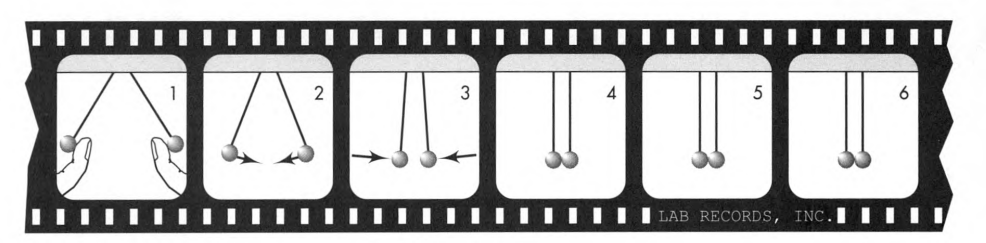
For system energy the outcome is more perplexing. Just before the collision, each ball has an energy consisting of its mass \(m\) and its kinetic energy \(K\). These energies add to make the total energy of the system: \(E_{\text {system }}=2 m+2 K\).
Kinetic energy converted to mass
After the collision? Both balls of chewing gum are at rest, stuck together as a single blob, which now constitutes the entire system. The energy of that stationary blob must be its rest energy, equal to the mass of the system: \(E_{\text {system }}=E_{\text {rest }}=M_{\text {system. }}\). What is the value of that system energy? It must be the same as the energy of the system before the collision, equal to \(2 m+2 K\), where \(m\) is the mass of each ball before the collision. Hence, if energy is conserved, \(M_{\text {system }}=2 m+2 K\). This is greater than the sum of masses of the incoming particles.
Where does this extra mass come from? The energy of relative motion of the incoming particles gets converted, during the collision, into energy of plastic deformation and heat. Each of these forms of locked-in energy yields an increment of mass. In consequence the mass of the pair of balls, stuck together as one, exceeds the sum of masses of the two balls before impact.
Third Experiment: Weighing Heat. If warmed and distorted balls of gum have more mass than cool and undistorted balls, then maybe we can measure directly the increased mass simply by heating an object and weighing it. In this case the system consists of a single large object, such as a tub of water, stationary and therefore with zero total momentum. System energy consists of the summed individual masses of all water molecules plus the summed kinetic energies of their random motions. This summed kinetic energy increases as we add heat to the water; hence its mass should increase. Can we detect the corresponding increase in weight as we heat the water in the tub?
Can we weigh heat? Not yet!
Alas, never yet has anyone succeeded in weighing heat. In 1787 Benjamin Thompson, Count Rumford (1753-1814), tried to detect an increase in weight of barrels of water, mercury, and alcohol as their temperature rose from \(29^{\circ} \mathrm{F}\) to \(61^{\circ} \mathrm{F}\) (in which range ice melts). He found no effect. He concluded "that ALL ATTEMPTS TO DISCOVER ANY EFFECTS OF HEAT UPON THE APPARENT WEIGHTS OF BODIES WILL BE FRUITLESS’ (capital letters his). Professor Vladimir Braginsky of the University of Moscow once described to us a new idea for weighing heat. Let a tiny quartz pellet hang on the end of a long thin near-horizontal quartz fiber, like a reeled-in fish at the end of a long supple fishing rod. A fly that settles on the fish increases its weight; the fishing rod bends a little more. Likewise heat added to the pellet will increase its mass and will bend the quartz-fiber "fishing rod" a little more. That is the idea. The sensitivity required to detect a bending so slight unfortunately surpasses the present limit of technology. Braginsky himself already has invented, published, and made available to workers all over the world a now widely applied scheme to measure very small effects. There is real hope that he-or someone else - will weigh heat and confirm what we already confidently expect.