6.1: Galileo Explores Gravity with Pendulums
- Page ID
- 31559
Legend has it that a young Galileo observed the swinging of a censer in church one day and noted that the incense burners kept swinging in time with each other as long as the chains that held them were of the same length. Galileo constructed his own pendulums and continued to experiment with them for much of his life. Like Galileo, we have much to learn from a swinging weight on the end of a length of string!
Science and Engineering Practices
- Planning and carrying out investigations.
- Analyzing and interpreting data.
- Argument from evidence.
Crosscutting Concepts
- Cause and effect.
- Structure and function.
Next Generation Science Standards
- Forces and interactions (K-5, 6-8, 9-12).
- Structure and function (K-5, 6-8, 9-12).
- Engineering and design (K-5, 6-8, 9-12).
- Gravitation and orbits (6-8, 9-12).
For the Educator
Facts you need to know
- Everything has its own gravity and gravity is always attractive. (Newton’s law of gravity.)
- Gravity is a property of mass; the more mass an object has, the more powerful its gravitational force will be. (Newton’s law of gravity.)
- Gravity makes everything fall at the same rate, but we can reduce gravity’s force, slow it down if you will, by using a ramp or a pendulum. (Galileo)
- Gravity is created when massive objects like planets pull on and stretch spacetime. (Einstein’s theory of general relativity.)
Teaching and Pedagogy
K-8 educators often shy away from topics like gravitation because they feel like the mathematics required will be beyond their younger student’s grasp. While this may be true, there is no reason to avoid topics like gravity which can be explored conceptually with low-cost, hands-on activities.
The key to making such activities successful in the classroom is an active guidance by the teacher that points out key ideas. Students, like everyone else, often see things all the while missing the important facts and ideas. Pointing out these ideas is the teacher’s role here; active guidance also helps students avoid forming misconceptions as they explore new activities.
Linking accurate observations with key ideas and explanations is a critical role for the educator. As a classroom teacher, you don’t need a mathematically sophisticated understanding of astronomy and physics to conduct successful science activities. You do need to understand a few key facts and ideas, and be able to recognize them and point them out as your students explore and learn.
Student Outcomes
What will the student discover?
- How heavy something makes no difference in how fast it falls. This was very confusing to ancient scientists, and still is to many modern people as well! Aristotle taught that the heavier a thing is, the faster it falls. It was Galileo who first proved Aristotle wrong and showed that all things fall at the same rate.
- It is the length of the string, not the weight on the end of it which controls how long it takes for a pendulum to swing back and forth.
- The way a weight swings on a string is intimately connected with the way that all things fall.We will explore this further in future activities.
What will your students learn about science?
- Old and established ideas are not necessarily true. We do not owe an idea respect or reverence simply because it is old, but we don’t just throw out ideas because they are old, either! Experimental evidence always trumps tradition.
- There are often subtle connections in science. The idea that a weight on a pendulum string is actually fallingas it swings is both powerful and subtle. Scientists and mathematicians had used and observed pendulums for thousands of years before Galileo discovered this important connection.
- Often, we can see and describe a pattern before we can understand it mathematically. In this case, our students will be exploring gravity in a powerful conceptual way to prepare them for the mathematical explanations they will be exposed to in years to come.
Conducting the Activity
Materials
- A ball of string, sturdy thread, or twine
- 20+ 3/8” metal washers to serve as weights
- Several large paper clips
- Wooden or plastic ruler
- A timer (a stopwatch app on a smart phone works well!)
- An 18-inch long flat board. This can be almost anything from a piece of sturdy cardboard, to a 2×4, a piece of shelving…
- [Optional] Three cup-hooks (the sort that hold up coffee cups beneath a shelf)
- Several rocks of various sizes, from fingernail size to chicken-egg sized.
Building the Pendulum Model:
- [Teacher] Securely screw three cup hooks into one side of your flat board. If you are using a piece of sturdy cardboard (triple thickness of copy paper box glued together with white glue does well!), you should use hot glue or super glue to make sure the hooks will not slip out! The board can now be placed across the backs of two chairs so that pendulum weights attached to the hooks can swing freely.
- Thread some washers onto three paper clips. 2 washers for the pendulum-A, 5 washers for pendulum-B, and 8 washers for pendulum-C. Tie a 2-ft length of string to each paper clip.
Exploring the Pendulum Model
- Now ask the class which pendulum will swing the fastest and which the slowest? Ask they to write down their answers and rate them 1-2-3.
- Now it is time to test our predictions! Secure all three strings to the hooks on the board. The exact length is not important, but use a ruler to insure that all three strings are the same length. Knot the strings securely and trim off any extra string with a scissors.
- With the board held steady, use the ruler to pull back the weights just a few inches and then release them together as the timer starts. Assign students to count how many complete swings out and back each pendulum makes. The timer lets the clock run for 5 seconds and then calls “STOP!”
- Note: Do not pull the pendulums back too far – this will interfere with good results. Pulling back 4-inches at most will work best.
- Most students will be amazed that the light, medium, and heavy weight pendulums all swing at the same rate! Weight has nothing to do with the speed of a pendulum! More on this in a bit!
- Ask your students to figure out a way for three pendulums to swing as different speeds. It probably won’t take long for someone to cry out: “Try different lengths!” Redo the pendulums so they all have the same number of washers (5 works well). Change the string on them so you have one short, one medium, and one long pendulum.
- Ask the students to think and record their predictions on a piece of paper again. It won’t take long for them to see that the shortest pendulum races along, while the longest moves at the slowest pace. Length is the crucial factor in pendulum time!
Discussion Questions
- What was the most surprising thing you learned about pendulums today?
- Answer Most students are amazed that weight has nothing to do with how fast a pendulum swings. Aristotle’s misconception that heavy things must fall faster than lighter ones is still alive and well today!
- Why are Grandfather Clocks so tall? (Look up an image of one on the internet!)
- Answer The pendulum on a Grandfather clock is about 2-feet long, this isn’t a coincidence! At this size, each swing of the pendulum from one side of the clock to another takes 1 second. The size of the clock is controlled by Earth’s gravity!
- How would you have to change the design of a grandfather clock if you were to build one on the Moon?
- Answer In the Moon’s 1/6th gravity, things fall much more slowly, and pendulums swing more slowly as well. In order to make a clock that tick-tocked once per second, the pendulum would have to be much shorter. A grandfather clock on the Moon would be only about 18-inches tall!
- What is the main thing that pendulums tell us about gravity?
- Answer Because a pendulum swings at the same speed no matter how large the weight is, this tells us that everything must fall at the same speed regardless of weight! Galileo referred to this property of matter as inertia. We will come at this idea again in Activity #16.
Supplemental Materials
Going Deeper
History tells us that Galileo first noticed the relationship between pendulum length and period (the time it takes for one swing out and back again) by watching and incense censer being swung back and forth during a church service. Galileo went on to investigate gravity with pendulums, ramps, even by dropping various iron weights off the leaning tower in Pisa, Italy!
Our exploration of gravity and pendulums is a simple (and surprising!), event for students. But why doesn’t weight cause the time of the pendulum to change? Don’t large weights experience more gravitational force than small ones? Shouldn’t the larger gravitational force cause them to go faster than the lighter weights? Surprisingly, the answer is no!
Galileo realized that although larger weights experience more gravitational force, they are also harder to move – Galileo called this property of matter inertia. You can try it yourself with a couple of rocks. Take a small rock, perhaps one inch across and see how far you can throw it. Now choose a larger rock, say 4 inches wide and see how far you can throw that! Your arm is just as strong, but inertia makes it harder to get the large rock moving so you cannot throw it as fast – or as far. If you are leery of trying to have your students throw rocks in school (really!?), have them try and throw a rubber T-ball and a basketball on the athletic field.
For our pendulums, it is much the same. The greater weight of the largest pendulum means it is pulled down with much more gravitational force than the lighter pendulum experiences. But the larger pendulum is also harder to move – the force of gravity and the pendulum weight’s inertia balance out exactly, and so the period of the pendulum remains the same as long as the length is the same. For our grandfather clock, a pendulum length of 50 cm (20 inches) makes the pendulum tick-tock gracefully with a two second period; one second to swing out, and one second to swing back. A grandfather clock pendulum on the Moon would be much shorter, just 8.2 cm long, because the Moon’s gravity is 1/6th that of Earth. On Jupiter, where the gravity is almost 4x that of Earth, a grandfather clock pendulum would have to be 2 meters long, that’s almost 7 feet!
Being an Astronomer
One of Isaac Newton’s great rivals was fellow British scientist Robert Hooke. Newton had perhaps the greatest mind ever for developing far reaching and sophisticated mathematical models and theories, but Robert Hooke was by far the more practical of the two men. Science needs both types!
After Newton had published his theory of universal gravitation that mathematically demonstrated both how and why planets orbit the Sun in neat elliptical pathways, Robert Hooke upstaged Newton at a meeting of the Royal Society (the British Academy of Science and Mathematics) by demonstrating what is called Hooke’s Pendulum, a simple and effective demonstration of orbital motion that you can reproduce in your classroom today.
While Newton had used many pages of complex and sophisticated mathematics using algebra, trigonometry, and calculus proving that planetary orbits were caused by the gravitational attraction of the Sun, Hooke used a simple mechanical model to demonstrate the same effect in seconds using no math at all! Everyone who saw it understood it almost instantly – your students will too!
Being a Scientist
Calculating the period, or cycle time of a pendulum is not terribly difficult; it can be done easily with most any school calculator. It is often interesting, and productive, for the gifted student to grapple with mathematical explanations rather than simply sticking to conceptual ideas.
The formula for the period of a pendulum involves only three numbers, and only one of these needs to be measured. For the purpose of our calculation, we needn’t worry about units. Our answer will automatically come out in seconds.
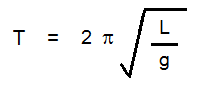
- We use T to represent the time or period needed for a pendulum to swing all the way out and back again. This is measured in seconds.
- We use L to measure the length of the pendulum. This is just the length of the string and is measured in meters. If you use measure the string in centimeters, divide your answer by 100 to convert the value into meters.
- π (or pi) is the ratio of a circles diameter to its circumference. This number never changes: π = 3.14
- g is used to represent the gravitational constant for the Earth. This is the rate at which things fall when we drop them – it applies to all objects, regardless of size, weight, or shape. g = 9.81 m/s2.
- Begin by taking the Length and divide by 9.81. Write this answer down.
- Take the square root of the first answer, write this value down.
- Multiply the second answer by 2, and then multiply again by 3.14.
- This final answer should be the period of your pendulum in seconds. Measure the period of your pendulum with a stop watch and see how close you get!
- Let’s try an example. Let’s say your string is 25 cm long (that’s 0.25 meters!). You calculate the period like this:
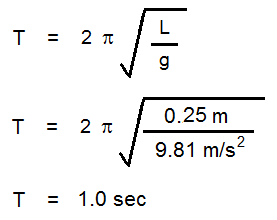
Following Up
Pendulums are used in many kinds of devices, from scientific and time keeping instruments to musical instruments. Besides the types of pendulums that swing on a string, essentially anything that vibrates back and forth can be considered a pendulum.
A guitar string vibrates back and forth when it is plucked, this back and forth motion is much the same as a clock pendulum – and the mathematics that governs the behavior of vibrating strings and pendulums is much the same. The wings of a fly, even the musical note we make when we blow across the mouth of a partially filled bottle are examples of vibrating pendulums in nature. How many can you think of?