1.3: Lipid Tails and Saturation
- Page ID
- 866
\( \newcommand{\vecs}[1]{\overset { \scriptstyle \rightharpoonup} {\mathbf{#1}} } \)
\( \newcommand{\vecd}[1]{\overset{-\!-\!\rightharpoonup}{\vphantom{a}\smash {#1}}} \)
\( \newcommand{\id}{\mathrm{id}}\) \( \newcommand{\Span}{\mathrm{span}}\)
( \newcommand{\kernel}{\mathrm{null}\,}\) \( \newcommand{\range}{\mathrm{range}\,}\)
\( \newcommand{\RealPart}{\mathrm{Re}}\) \( \newcommand{\ImaginaryPart}{\mathrm{Im}}\)
\( \newcommand{\Argument}{\mathrm{Arg}}\) \( \newcommand{\norm}[1]{\| #1 \|}\)
\( \newcommand{\inner}[2]{\langle #1, #2 \rangle}\)
\( \newcommand{\Span}{\mathrm{span}}\)
\( \newcommand{\id}{\mathrm{id}}\)
\( \newcommand{\Span}{\mathrm{span}}\)
\( \newcommand{\kernel}{\mathrm{null}\,}\)
\( \newcommand{\range}{\mathrm{range}\,}\)
\( \newcommand{\RealPart}{\mathrm{Re}}\)
\( \newcommand{\ImaginaryPart}{\mathrm{Im}}\)
\( \newcommand{\Argument}{\mathrm{Arg}}\)
\( \newcommand{\norm}[1]{\| #1 \|}\)
\( \newcommand{\inner}[2]{\langle #1, #2 \rangle}\)
\( \newcommand{\Span}{\mathrm{span}}\) \( \newcommand{\AA}{\unicode[.8,0]{x212B}}\)
\( \newcommand{\vectorA}[1]{\vec{#1}} % arrow\)
\( \newcommand{\vectorAt}[1]{\vec{\text{#1}}} % arrow\)
\( \newcommand{\vectorB}[1]{\overset { \scriptstyle \rightharpoonup} {\mathbf{#1}} } \)
\( \newcommand{\vectorC}[1]{\textbf{#1}} \)
\( \newcommand{\vectorD}[1]{\overrightarrow{#1}} \)
\( \newcommand{\vectorDt}[1]{\overrightarrow{\text{#1}}} \)
\( \newcommand{\vectE}[1]{\overset{-\!-\!\rightharpoonup}{\vphantom{a}\smash{\mathbf {#1}}}} \)
\( \newcommand{\vecs}[1]{\overset { \scriptstyle \rightharpoonup} {\mathbf{#1}} } \)
\( \newcommand{\vecd}[1]{\overset{-\!-\!\rightharpoonup}{\vphantom{a}\smash {#1}}} \)
\(\newcommand{\avec}{\mathbf a}\) \(\newcommand{\bvec}{\mathbf b}\) \(\newcommand{\cvec}{\mathbf c}\) \(\newcommand{\dvec}{\mathbf d}\) \(\newcommand{\dtil}{\widetilde{\mathbf d}}\) \(\newcommand{\evec}{\mathbf e}\) \(\newcommand{\fvec}{\mathbf f}\) \(\newcommand{\nvec}{\mathbf n}\) \(\newcommand{\pvec}{\mathbf p}\) \(\newcommand{\qvec}{\mathbf q}\) \(\newcommand{\svec}{\mathbf s}\) \(\newcommand{\tvec}{\mathbf t}\) \(\newcommand{\uvec}{\mathbf u}\) \(\newcommand{\vvec}{\mathbf v}\) \(\newcommand{\wvec}{\mathbf w}\) \(\newcommand{\xvec}{\mathbf x}\) \(\newcommand{\yvec}{\mathbf y}\) \(\newcommand{\zvec}{\mathbf z}\) \(\newcommand{\rvec}{\mathbf r}\) \(\newcommand{\mvec}{\mathbf m}\) \(\newcommand{\zerovec}{\mathbf 0}\) \(\newcommand{\onevec}{\mathbf 1}\) \(\newcommand{\real}{\mathbb R}\) \(\newcommand{\twovec}[2]{\left[\begin{array}{r}#1 \\ #2 \end{array}\right]}\) \(\newcommand{\ctwovec}[2]{\left[\begin{array}{c}#1 \\ #2 \end{array}\right]}\) \(\newcommand{\threevec}[3]{\left[\begin{array}{r}#1 \\ #2 \\ #3 \end{array}\right]}\) \(\newcommand{\cthreevec}[3]{\left[\begin{array}{c}#1 \\ #2 \\ #3 \end{array}\right]}\) \(\newcommand{\fourvec}[4]{\left[\begin{array}{r}#1 \\ #2 \\ #3 \\ #4 \end{array}\right]}\) \(\newcommand{\cfourvec}[4]{\left[\begin{array}{c}#1 \\ #2 \\ #3 \\ #4 \end{array}\right]}\) \(\newcommand{\fivevec}[5]{\left[\begin{array}{r}#1 \\ #2 \\ #3 \\ #4 \\ #5 \\ \end{array}\right]}\) \(\newcommand{\cfivevec}[5]{\left[\begin{array}{c}#1 \\ #2 \\ #3 \\ #4 \\ #5 \\ \end{array}\right]}\) \(\newcommand{\mattwo}[4]{\left[\begin{array}{rr}#1 \amp #2 \\ #3 \amp #4 \\ \end{array}\right]}\) \(\newcommand{\laspan}[1]{\text{Span}\{#1\}}\) \(\newcommand{\bcal}{\cal B}\) \(\newcommand{\ccal}{\cal C}\) \(\newcommand{\scal}{\cal S}\) \(\newcommand{\wcal}{\cal W}\) \(\newcommand{\ecal}{\cal E}\) \(\newcommand{\coords}[2]{\left\{#1\right\}_{#2}}\) \(\newcommand{\gray}[1]{\color{gray}{#1}}\) \(\newcommand{\lgray}[1]{\color{lightgray}{#1}}\) \(\newcommand{\rank}{\operatorname{rank}}\) \(\newcommand{\row}{\text{Row}}\) \(\newcommand{\col}{\text{Col}}\) \(\renewcommand{\row}{\text{Row}}\) \(\newcommand{\nul}{\text{Nul}}\) \(\newcommand{\var}{\text{Var}}\) \(\newcommand{\corr}{\text{corr}}\) \(\newcommand{\len}[1]{\left|#1\right|}\) \(\newcommand{\bbar}{\overline{\bvec}}\) \(\newcommand{\bhat}{\widehat{\bvec}}\) \(\newcommand{\bperp}{\bvec^\perp}\) \(\newcommand{\xhat}{\widehat{\xvec}}\) \(\newcommand{\vhat}{\widehat{\vvec}}\) \(\newcommand{\uhat}{\widehat{\uvec}}\) \(\newcommand{\what}{\widehat{\wvec}}\) \(\newcommand{\Sighat}{\widehat{\Sigma}}\) \(\newcommand{\lt}{<}\) \(\newcommand{\gt}{>}\) \(\newcommand{\amp}{&}\) \(\definecolor{fillinmathshade}{gray}{0.9}\)Lipids are molecules that are composed of a hydrophilic head group and a hydrophobic tail. Lipid bilayers form to remove the hydrophobic tails from the aqueous phase. The lipid tails face the interior of a biological membrane (Figure \(\PageIndex{1}\)). Phospholipids, glycerolipids, and sphingolipids contain one or more (usually two) fatty acid chains as hydrophobic tails. A portion of the sphingoid base also makes up part of the hydrophobic tail of a sphingolipid. The hydrophobic tail dominates the size of the structure of sterols, excluding the hydroxl group. The shape and size of the lipid tail contribute significantly to the physical properties of the membrane.

Fatty acids
The majority of membrane lipid tails are made up of esterified fatty acids. This means that the carboxylic acid group forms a bond with the glycerol backbone resulting in an ester group. The hydrophobic tails consists of fatty acids. Fatty acids vary in both chain length and degree of saturation. The chain length is the number of carbons in the fatty acid. The fatty acids can be completely saturated, meaning the carbons are all connected by single bonds, or unsaturated, meaning the chain contains one or more double bonds. A given fatty acid can be named in three ways based off of their chain length and degree of saturation. First, many have common, or trivial names. For example, a 16 carbon fully saturated fatty acid is palmitic acid. An 18 carbon fatty acid with one cis double bond at the 9th position is oleic acid. Another naming system is the shorthand structural. This consists of 2 numbers separated by a colon. The first number is the number of carbons in the chain while the second is the number of double bonds. Additional numbers are added after a delta (Δ) symbol to indicate the positions of the double bonds. Since most biologically occurring unsaturated fatty acid have cis double bonds, there is nothing added after the number to signify cis. However, if the double bond is trans, the number will be followed by a “t”. Palmitic acid and oleic acid are thus 16:0 and 18:1Δ9 respectively (Sitwell 2013). Lastly, there is the system of nomenclature supported by IUPAC and IUBMB. In this system, the fatty acid takes its carboxylic acid name. A 16 carbon saturated fatty acid is hexadecanoic acid. An 18 carbon mono-unsaturated at the 9th position fatty acid is 9Z-octadecenoic acid. The “9Z” at the beginning specifies the location of the double bond and that it is cis. When naming a phospholipid or glycerolipid containing fatty acid tails, the name of the tails precede the name of the headgroup. Since the fatty acids are esterified, the suffix is changed from -oic to -oyl. The systematic name for a common phospholipid is 1-hexadecanoyl-2-(9Z-octadecenoyl)-sn-glycero-3-phosphocholine (Fahy et al 2011). This has hexadecanoic acid at position 1 and 9Z-octadecenoic acid at position 2 of the glycerol backbone (Figure \(\PageIndex{2}\)A). Fatty acids can also be given an omega designation which is determined by the position of the double bond carbon nearest to the methyl end. An omega 3 fatty acid is one were the 3rd and 4th carbons from the methyl are connected by a double bond (Uttaro 2008).
Glycerophospholipids
The most common type of membrane lipids are glycerophospholipids or glycerolipids. Phosphoglycerides are prominent in higher animals (such as vertebrates) while glycosylglycerides are important in plants. There are three chief classes of membrane lipid which are widely distributed- glycerolipids, sphingolipids, and steroids. Glycerolipids, related to their abundance, are the most important group. They can be divided into two main groups – phosphoglycerides (containing phosphorus) and glycosylglycerides (without phosphorus but containing a sugar constituent) Phosphoglycerides are the major lipid components of most biological membranes. Phosphoglycerides consist of a diverse and vast array of structures. They are the primary lipid components in most membranes, excluding photosynthetic membranes of plants, algae, and cyanobacteria as well as archaebacterial membranes. Typically, phosphoglycerides contain fatty acids esterified at positions 1 and 2 of glycerol making them diacylphosphoglycerides. The names of these lipids come from the component which is attached to the phosphate esterified at position 3 of glycerol. Therefore, the compounds are essentially derivatives of diacylglycerols in which the hydroxyl on the carbon 3 atom is esterified with phosphoric acid. Moreover, the simplest phosphoglyceride contains only phosphoric acid attached to diacylglycerol which is called phosphatidic acid. (Gurr, 2005)
Glucosylglycerides are predominant components of photosynthetic membranes and play major role in some micro-organisms. Their structure is comparable to that of glycerophospholipids with the sugars attached glycosidically to position 3 of glycerol and fatty acids esterified at the other two positions. Roughly 40% of the dry weight of photosynthetic membranes of higher plants consists of two galactose-containing lipids – monogalactosyldiacylglcerol and digalactosyldiacylglycerol. There is a β-link in the first position of the galactose ring to glycerol while in digalactosyldiacylglycerol there is an α, 1,6-bond between sugars. Many combinations of residues in diglycosyldiacylglycerols can be found in bacteria-most abundantly are two glucose, two galactose, or two mannose residues linked α,1-2 or β,1-6.
Aside from galactose-containing lipids, a third glycosylglyceride, plant sulpholipid, is found in chloroplasts. It is more formally referred to as sulphoquinovosyliacylglycerol and it contains a sulphonate constituent on carbon 6 of a deoxyglucose residue. This is a naturally occurring negatively charged molecule with a very stable sulphonic acid group. This sulpholipid is highly characteristic of the photosynthetic membranes of chloroplasts and cyanobacteria. All of the chloroplast glycolipids usually consists of great amounts of α-linolenic acid. Moreover, monogalactosyldiacylglycerol may have up to 97%of its total acyl groups as this one component in some plants. (Gurr,2005)
Sterols
Since the hydrophobic tail of a sterol is essentially a steroid, it is named accordingly. The suffix -ol is added to designate that it is a sterol (Figure \(\PageIndex{2}\)B).

Many sterols are significant units of many organisms especially their external cellular membranes. However, sterols are not necessary or crucial components by all organisms even organisms with significant sterol content. Sterols are widespread in eurkaryotes, but are rare in prokaryotes. Yeast and fungi have side chain alkylated compounds while plants and algae contain sitosterol and stigmasterol as their most com common sterols. The primary function for membrane sterol is the modulation of fluidity which is resolved by the interaction of sterol with the glycerolipid components.
Sphingolipids
The hydrophobic tails of sphingolipids are made up of a fatty acid and a long carbon chain on the sphingoid base. The name of the lipid is thus a combination of the name of the sphingoid base and the fatty acid (Figure \(\PageIndex{2}\)C) (Fahy et al 2011).
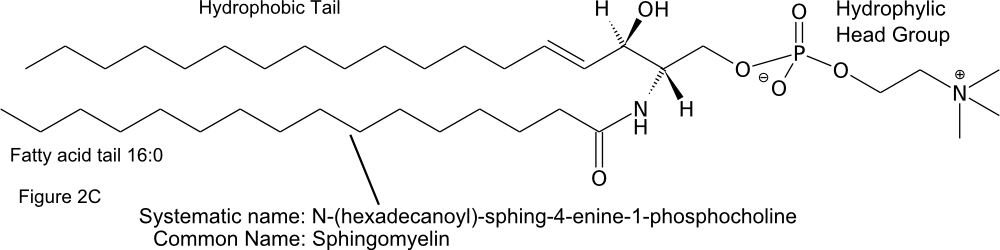
Fatty Acid Biosynthesis
Fatty acid synthesis pathways are placed in two classes, type I and II. The steps in the type II pathway are catalyzed by separate enzymes. In type I, multienzymes contain multiple domains that catalyze several steps in the synthesis pathway. Type II is found in eubacteria and organelles of prokaryotic origin (mitochondria and plastids) while Type I is found in eukaryotes (Schweizer and Hofmann 2004). Although the enzyme organization is different, the basic steps of fatty acid synthesis are conserved across bacteria and eukaryotes and involve the sequential addition of acetyl groups from acetyl-coA to a growing fatty acid chain. To produce saturated fatty acids addition of acetyl groups proceeds to form a chain of usually 16 or 18 carbons (Figure \(\PageIndex{3}\)).
\[ 8 \text{Acetyl-coA} + 7\text{ATP} + 14\text{NADPH} \rightarrow \text{Palmitic Acid} + 7\text{ADP} + 7\ce{P_i} + 14\text{NADP} + 6 \ce{H2O} + 8 \text{coA}\]
Synthesis of an unsaturated fatty acid in bacteria occurs in a similar manner to saturated fatty acid synthesis up to the 10 carbon intermediate. At this point a double bond is formed between the 3rd and 4th carbons on the chain (numbering from the side of addition). After the cis double bond is added, the addition of acetyl units continues to 16 or 18 carbons, meaning the double bond will be at positions 9 and 11 respectively (Chan and Vogel 2010). In eukaryotes, double bonds are added after fatty acid synthesis. The enzymes that produce mono-unsaturated fatty acids preferentially introduce a double bond between carbons 9 and 10. This means that 16:1Δ9 and 18:1Δ9 are common on lipid tails. Another set of enzymes adds double bonds near the methyl end of already unsaturated fatty acids. These preferentially produce bonds between the 3rd and 4th carbons and between the 6th and 7th carbons from the methyl end (omega 3 and 6). Mammals do not have the enzymes needed to add double bonds near the methyl end. Additional double bonds are added near the carboxyl end of fatty acids that already contain double bonds added by the other two enzyme classes (Uttaro 2008). Thus, the degree of unsaturation and placement of the double bonds is determined by these enzymes.
Two fatty acid chains are attached to positions 1 and 2 of glycerol-3-phosphate to form phospatidic acid (Figure \(\PageIndex{4}\)). This is later converted to several other lipids by addition of diverse head groups . Some bacteria are able to introduce double bonds to the fatty acid chain after the lipid is formed (Zhang and Rock 2008).
Effects on membranes
Membrane fluidity and phase: The fluidity of a biological membrane is related to the melting temperature of the lipid tails within it. The melting temperature is affected by both the chain length and saturation of the tail. Longer chains lead to higher melting temperature because they are able to form stronger van der Waals forces between molecules. Higher degrees of unsaturation lead to lower melting temperature. This is because the double bonds add kinks to the fatty acid chains which prevent tight packing of lipid molecules. In general, the degree of saturation impacts the fluidity of the membrane to a greater extent than chain length (Sitwell 2013). Membranes of single lipid composition exist in two phases. At high temperatures they are in a highly fluid liquid-crystalline phase. At low temperatures they are at a rigid gel phase. In membranes that contain cholesterol, the gel phase is replaced by a liquid-ordered phase that maintains some fluidity (Komura and Andelman 2014). Lipids with saturated tails are prone to the liquid-ordered phase, while lipids with unsaturated tails are prone to the liquid-crystalline, or liquid-disordered phase. In the liquid-ordered phase, the carbon-carbon bonds maintain trans-rotomers, meaning the tails straighten out as much as possible. The width of the hydrophobic portion of lipids with long saturated tails will be greater than those with shorter or unsaturated tails because double bonds can prevent the tails from straightening out completely and packing closely. The difference in the hydrophobic width can lead to hydrophobic mismatch and phase separation (Komura and Andelman 2014).

Interactions with membrane proteins: The hydrophobic lipid tails are responsible for solublizing the trans-membrane portions of a membrane protein within the membrane which illustrates one of the their many roles. A ring of lipids, referred to as a lipid annulus, surrounds the protein and the relatively flexible tails cover the surface of protein (Lee 2011). Properties of the membrane conferred by the lipid tail, such as the thickness of the hydrophobic phase, compressibility, and intrinsic curvature can significantly impact protein folding and function within the membrane (Andersen et al 2007).
Citations
- Stillwell, William. An Introduction to Biological Membranes: From Bilayers to Rafts. Chapter 4 - Membrane Lipids: Fatty Acids. Amsterdam: Elsevier/Academic, 2013. pg 43-56
- Fahy E, Cotter D, Sud M, Subramaniam S. Lipid classification, structures and tools. Biochim Biophys Acta. 2011;1811(11):637-47.
- Uttaro AD. Biosynthesis of polyunsaturated fatty acids in lower eukaryotes. IUBMB Life. 2006;58(10):563-71.
- Schweizer E, Hofmann J. Microbial type I fatty acid synthases (FAS): major players in a network of cellular FAS systems. Microbiol Mol Biol Rev. 2004;68(3):501-17
- Chan DI, Vogel HJ. Current understanding of fatty acid biosynthesis and the acyl carrier protein. Biochem J. 2010;430(1):1-19.
- Zhang YM, Rock CO. Membrane lipid homeostasis in bacteria. Nat Rev Microbiol. 2008;6(3):222-33.
- Komura S, Andelman D. Physical aspects of heterogeneities in multi-component lipid membranes. Adv Colloid Interface Sci. 2014;208C:34-46.
- Shibata Y, Hu J, Kozlov MM, Rapoport TA. Mechanisms shaping the membranes of cellular organelles. Annu Rev Cell Dev Biol. 2009;25:329-54.
- Andersen OS, Koeppe RE. Bilayer thickness and membrane protein function: an energetic perspective. Annu Rev Biophys Biomol Struct. 2007;36:107-30.
- Sud M, Fahy E, Cotter D, Brown A, Dennis EA, Glass CK, Merrill AH Jr, Murphy RC, Raetz CR, Russell DW, Subramaniam S. LMSD: LIPID MAPS structure database Nucleic Acids Research 35: p. D527-32.
- GChemPaint Chemical Structures Editor. http://savannah.nongnu.org/projects/gchempaint/
- Gurr, M. I., J. L. Harwood, and K. N. Frayn. Lipid Biochemistry. Oxford, UK: Blackwell Science, 2005
Contributors and Attributions
- Philip Day (UC Davis) and William Scott (UC Davis)