1.2: Lipid Headgroup Types
- Page ID
- 864
\( \newcommand{\vecs}[1]{\overset { \scriptstyle \rightharpoonup} {\mathbf{#1}} } \)
\( \newcommand{\vecd}[1]{\overset{-\!-\!\rightharpoonup}{\vphantom{a}\smash {#1}}} \)
\( \newcommand{\id}{\mathrm{id}}\) \( \newcommand{\Span}{\mathrm{span}}\)
( \newcommand{\kernel}{\mathrm{null}\,}\) \( \newcommand{\range}{\mathrm{range}\,}\)
\( \newcommand{\RealPart}{\mathrm{Re}}\) \( \newcommand{\ImaginaryPart}{\mathrm{Im}}\)
\( \newcommand{\Argument}{\mathrm{Arg}}\) \( \newcommand{\norm}[1]{\| #1 \|}\)
\( \newcommand{\inner}[2]{\langle #1, #2 \rangle}\)
\( \newcommand{\Span}{\mathrm{span}}\)
\( \newcommand{\id}{\mathrm{id}}\)
\( \newcommand{\Span}{\mathrm{span}}\)
\( \newcommand{\kernel}{\mathrm{null}\,}\)
\( \newcommand{\range}{\mathrm{range}\,}\)
\( \newcommand{\RealPart}{\mathrm{Re}}\)
\( \newcommand{\ImaginaryPart}{\mathrm{Im}}\)
\( \newcommand{\Argument}{\mathrm{Arg}}\)
\( \newcommand{\norm}[1]{\| #1 \|}\)
\( \newcommand{\inner}[2]{\langle #1, #2 \rangle}\)
\( \newcommand{\Span}{\mathrm{span}}\) \( \newcommand{\AA}{\unicode[.8,0]{x212B}}\)
\( \newcommand{\vectorA}[1]{\vec{#1}} % arrow\)
\( \newcommand{\vectorAt}[1]{\vec{\text{#1}}} % arrow\)
\( \newcommand{\vectorB}[1]{\overset { \scriptstyle \rightharpoonup} {\mathbf{#1}} } \)
\( \newcommand{\vectorC}[1]{\textbf{#1}} \)
\( \newcommand{\vectorD}[1]{\overrightarrow{#1}} \)
\( \newcommand{\vectorDt}[1]{\overrightarrow{\text{#1}}} \)
\( \newcommand{\vectE}[1]{\overset{-\!-\!\rightharpoonup}{\vphantom{a}\smash{\mathbf {#1}}}} \)
\( \newcommand{\vecs}[1]{\overset { \scriptstyle \rightharpoonup} {\mathbf{#1}} } \)
\( \newcommand{\vecd}[1]{\overset{-\!-\!\rightharpoonup}{\vphantom{a}\smash {#1}}} \)
Lipid headgroups comprise part of the hydrophilic backbone of membrane phospholipids. There are a number of headgroups, each of which correlates with a particular type of backbone. Membrane lipids are split into three categories: phospholipids and glycolipids (Figure \(\PageIndex{1}\)). Sterols are the third type of membrane lipid (not pictured) that will be discussed.

Lipid headgroups are substituents that attach to the glycerol, sphingosine, or cholesterol backbones of polar membrane lipids. Phospho- and glycolipids have headgroups linked to the backbone through a phosphodiester bond (Figure \(\PageIndex{2}\)).
Understanding head group behavior is necessary for the study of membrane biology. The high structural diversity allows for a large range of functions, including effects on membrane curvature [1] cell signaling, substrate transport [2], and more. Consequently, their crucial role in membrane biology also ties them in with a broad range of diseases, from cardiovascular defects to cancer.
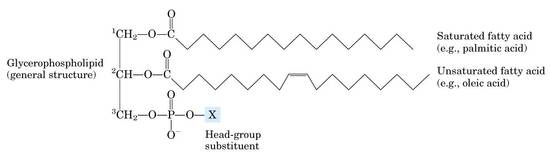
II. PHOSPHOLIPIDS
A. GLYCEROPHOSPHOLIPIDS
Glycerophospholipids are classified by the alcohol that is bound to the phosphate group on the glycerol backbone. The backbone always consists of a glycerol esterified to fatty acids in positions Csn-1 and Csn-2, with the head group substituent bound to the phosphate in position Csn-3 [2]. Interestingly, archaebacteria have the headgroup at the Csn-1 position [3]. The headgroup classes are differentiated by the Csn-1 and Csn-2 substituents. If the Csn-1 and Csn-3 substituents are unique, the Csn-2 carbon becomes a chiral center [4].
Phosphatidic acid has the simplest substituent: hydrogen. This parent compound, phosphomonoester, is then built upon by naming the derivatives for the head-group alcohol (X, Figure \(\PageIndex{2}\)) with “phosphatidyl-” as a prefix. For example, phosphatidyl-choline, phosphatidyl-ethanolamine, and phosphatidyl-serine are other common membrane lipids [4].
Some of the common glycerophospholipid head groups are shown in Table 1:

Phosphatidylinositol (PI) is interesting in that it can be phosphorylated to make PI phosphate (PIP), PI bisphosphate (PIP2), and PI trisphosphate (PIP3). These compounds are found on the cytoplasmic leaflet of membranes and therefore heavily influence the membrane-cytosol interface. This makes them particularly relevant to membrane trafficking, regulating cell growth, proliferation, and apoptosis [2].
B. SPHINGOLIPIDS
Sphingolipids have a base made of a long (carbon) chain called sphingosine, which is bound with fatty acids using amide linkages to make ceramides (Figure \(\PageIndex{3}\)). The sphingoid base backbone is synthesized from a long-chain fatty acyl-CoA and serine. Ceramide is the most basic sphingolipid, with only a hydrogen in the Csp-3 position and an amide-linked fatty acid [4] (Table 2). More complex (phosphor)sphingolipids have headgroups attached via phosphodiester linkages [4]. The Sphingosine backbone, fatty acid, and headgroup of a sphingolipid are also highlighted in Table 2.
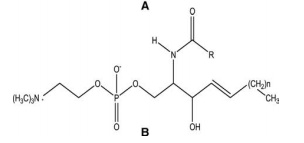
Some common sphingolipids headgroups are depicted in Table 2:

Sphingomyelin is a common sphingolipid that is prominent in the myelin sheaths of neurons. The head groups have a large effect on overall lipid structure, which is why phosphatidyl choline (gylerophospholipid) and sphingomyelin (sphingolipid) look so similar (Figure \(\PageIndex{4}\)).

Figure \(\PageIndex{4}\): Both phosphatidylcholine and sphingomyelin have a choline head group attached to a phosphate group. Despite the different backbone molecule, the two molecules are remarkably similar in shape. Source: Lafer, Eileen. “Membrane Lipids I and II: Glycerophospholipids and Sphingolipids.” Lecture. San Antonio, Texas. December 6, 2011
III. GLYCOLIPIDS
Glycolipids contain a sub-group of sphingolipids that have a similar backbone structure to phosphosphingolipids (Figure \(\PageIndex{3}\)), but have a mono- or oligosaccharide bound to the Csn-3 position instead of a phosphate-choline group (Table 2). Glycosphingolipids are especially relevant to neuronal membranes, where D-glucose is added to the hydroxyl group of ceramide to create GlcCer. If the headgroup has any (one to four) silica acid(s) attached to it, the new molecule is referred to as a ganglioside [2].
Interestingly, the carbohydrate head group portions of sphingolipids are what define the human blood groups. The oligosaccharide head groups are composed of D-glucose (Glc), D-galactose (Gal), N-acetyl-D-galactosamine (GalNAc), and fucose (Fuc). The addition of an extra GalNAc creates the A antigen/blood type, and the addition of a Gal creates the B antigen/blood type (Figure \(\PageIndex{5}\)).

STEROLS
Sterols in mammals stem primarily from cholesterol (Figure \(\PageIndex{6}\)) and its derivatives. Sterols are subdivided primarily based on biological function; steroids, a set of important hormones and signaling molecules [5] can also be subdivided by the number of carbons in the core skeleton. Estrogens fall into the C18 family, while androgens make up the C19 family [4]. A broad representation of sterols can be seen below (Figure \(\PageIndex{6}\)).

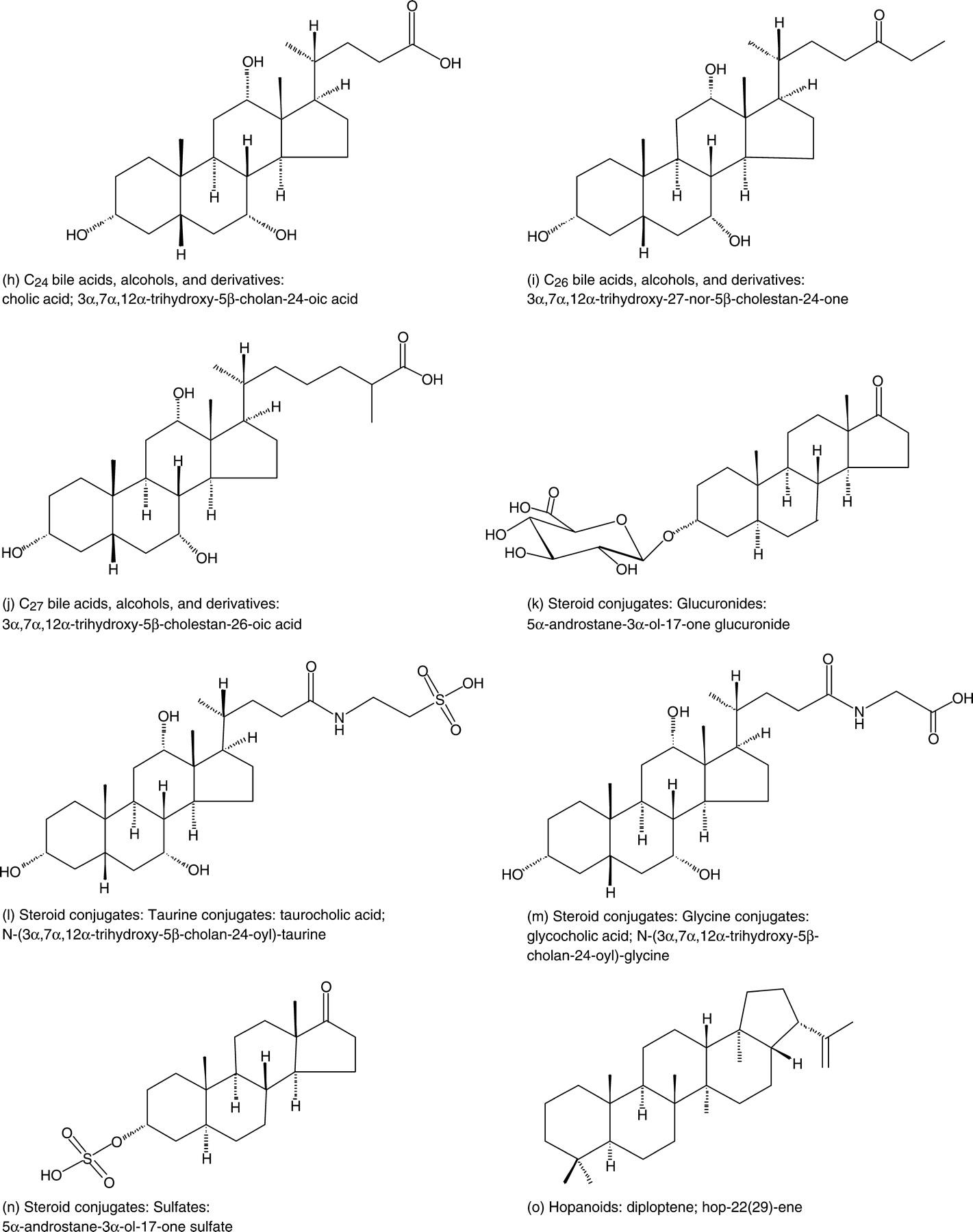
Figure \(\PageIndex{6}\): Representative structures for sterol lipids; source: Fahy E et al. J. Lipid Res. 2005;46:839-862, ©2005 by American Society for Biochemistry and Molecular Biology
CHARGED HEADGROUPS
Many lipids (both glycerophospholipids and sphingolipids) have charged headgroups. This charge is incredibly important in determining headgroup behavior, as it affects repulsion with other molecules and other membrane characteristics. For example, phospholipid headgroups interact with other membrane proteins like the P-glycoprotein multidrug transporter, which is linked to cancer resistance [6]. Similarly, charge density and headgroup structure influence membrane rigidity [7], create variation in protein-lipid interactions and liposome membrane mobility [8], and determine the ability of lipid-DNA complexes to transfect DNA in vitro [9].
There are many negatively charged headgroups, whereas positive charges are much less common. Phosphatidic acid is a dynamic headgroup that is uncharged below pH 4, but has a -1 charge at physiological (human) pH and a -2 charge above pH 14. Similarly, phosphatidylserine has a -1 charge and phosphatidylcholine is zwitterionic (Figure \(\PageIndex{7}\)).

The presence and concentration of charge has direct effects on headgroup orientation. If the electric surface charge of a membrane is positive, the N+ component of the P—N dipole (see phosphatidylcholine, Figure \(\PageIndex{7}\)) moves toward the water phase, which causes a large (>30˚) change in orientation of the phosphate segment. A negative surface charge has the opposite effect, moving the N+ end of the dipole toward the interior area of the membrane [10]. The orientation of lipids, and therefore the movement of headgroups, is important for lipid-protein binding and membrane topology. Finally, headgroup charge is notably related to membrane curvature. Studies have shown that the negatively charged headgroups phosphatidic acid and phosphatidylserine favor negative membrane curvature when excess ion are present (due to charge repulsion) and nearly flat surfaces in pure water. However, it is important to consider salt and pH conditions, as these may have an effect on the data [1, 11, 12].
CONCLUSION
In summary, there are three major membrane lipid groups: sterols, glycolipids, and phospholipids. These groups are determined based on the molecular backbone of the lipid. A variety of headgroups can then attach and alter structure and function. Many of these membrane lipids are directly associated with cellular processes, and therefore present health risks if perturbed. One case study showed that higher levels of plasma sphingolipids increases risk or occurence of lung cancer [13]. Headgroups also largely affect biological functions due to their ability to be charged, and often to change charge at different various pH values.
References
- Sodt, A.J. and R.W. Pastor, Molecular modeling of lipid membrane curvature induction by a Peptide: more than simply shape. Biophys J, 2014. 106(9): p. 1958-69.
- Montealegre, C., et al., Analysis of glycerophospho- and sphingolipids by CE. Electrophoresis, 2014. 35(6): p. 779-92.
- Pereto, J., P. Lopez-Garcia, and D. Moreira, Ancestral lipid biosynthesis and early membrane evolution. Trends Biochem Sci, 2004. 29(9): p. 469-77.
- Fahy, E., et al., A comprehensive classification system for lipids. J Lipid Res, 2005. 46(5): p. 839-61.
- Tsai, M.J. and B.W. O'Malley, Molecular mechanisms of action of steroid/thyroid receptor superfamily members. Annu Rev Biochem, 1994. 63: p. 451-86.
- Sharom, F.J., Complex Interplay between the P-Glycoprotein Multidrug Efflux Pump and the Membrane: Its Role in Modulating Protein Function. Front Oncol, 2014. 4: p. 41.
- Bruning, B., et al., Influence of charge density on bilayer bending rigidity in lipid vesicles: a combined dynamic light scattering and neutron spin-echo study. Eur Phys J E Soft Matter, 2013. 36(7): p. 77.
- Shimanouchi, T., et al., Relationship between the mobility of phosphocholine headgroup and the protein-liposome interaction: A dielectric spectroscopic study. Colloids Surf B Biointerfaces, 2014. 116: p. 343-50.
- Zhang, X.X., et al., Lipid-mediated DNA and siRNA Transfection Efficiency Depends on Peptide Headgroup. Soft Matter, 2013. 9(17).
- Scherer, P.G. and J. Seelig, Electric charge effects on phospholipid headgroups. Phosphatidylcholine in mixtures with cationic and anionic amphiphiles. Biochemistry, 1989. 28(19): p. 7720-8.
- Fuller, N., C.R. Benatti, and R.P. Rand, Curvature and bending constants for phosphatidylserine-containing membranes. Biophys J, 2003. 85(3): p. 1667-74.
- Kooijman, E.E., et al., Spontaneous curvature of phosphatidic acid and lysophosphatidic acid. Biochemistry, 2005. 44(6): p. 2097-102.
- Alberg, A.J. et al., Plasma sphingolipids and lung cancer: a population-based, nested case-control study.Cancer Epidemiol Biomarkers Prev. 2013. 22(8): p. 1374-82.