1.5: Sphingolipids
( \newcommand{\kernel}{\mathrm{null}\,}\)
Sphingolipids are a type of lipids made up of fatty acid chains that were first mentioned in 1884 in J.L.W. Thudichum’s A Treatise on the chemical composition of the brain. They were named after the Greek mythological creature, the sphinx, due to the unknown riddle of their function.[1] Sphingolipids are found in essentially all plants, animals, fungi and in some prokaryotes and viruses. Specifically, they are found in membranes and as a major component of lipoproteins.[2]
Structure
Sphingolipids are amphipathic molecules; they have hydrophobic and hydrophilic properties. In the hydrophobic region there is a sphingoid long chain base (aliphatic chains with attached hydroxyl groups) with a fatty acid chain attached by amide bond at carbon 2. In the hydrophilic region, there are phosphate groups, sugar residues, and/or hydroxyl groups.[3] This amphipathic nature allows for the diffusion of sphingolipids between membranes and for the flipping of sphingolipids between membrane leaflets. However, sphingolipids are still more likely to accumulate in acidic environments because of the possible ionization of a free amino group.[4] There are several classes of sphingolipids: the sphingoid base and simple derivatives, ceramides, and complex sphingolipids (Figure 1.5.1).

Sphingoid base & simple derivatives
Sphingolipids are composed of many backbone “sphingoid bases” which are synthesized from serine and a long-chain fatty acyl-CoA.[2,5] If a backbone has not yet been analyzed, it is referred to as a sphinosine, after the original name for the fundamental component of these lipids. Sphingoid bases can vary in alkyl chain length/branching, the number and position of double bonds, the number and locations of hydroxyl groups, etc. A simple sphinosine base can be seen in Figure 1.5.1.
These simple derivatives exist mainly as the backbone of more complex sphingolipids, but they do exist on their own. They are mainly used in intra-and extra-cellular signaling.[2] The exact structure of the sphingolipid determines the function. For example, in the dermis, there are additional hydroxyl groups at positions 4 and/or 6 that interact with neighboring molecules to strengthen the permeability of the skin barrier.[5]
Ceramides
Ceramides, which are synthesized in the endoplasmic reticulum, are the simplest sphingolipid after the backbone.[3] They are fatty acid derivatives of sphingoid bases, linked by amide bonds. They are usually fully saturated or mono-unsaturated and consist of 14-26 carbons, but are sometimes longer. There is sometimes a hydroxyl group on the ω or ω-carbon atom.[2,5] The structure can be seen in Figure 1.5.2. Ceramides have high phase transition temperatures of greater than 37°C. This is due to their predominately saturated nature, which allows for the tight packing of lipids. This also favors the segregation of ceramides (and more complex sphingolipids) into regions called membrane “rafts.”[2,6]
Ceramides are key to cell signaling processes.[3] They generally serve as second messengers by regulating cell growth, senescence, and apoptosis. The exact function depends on the type of sphingolipid base and fatty acid.[2] For example, ceramides containing palmitic acid for a fatty acid chain are generated in response to apoptosis. Much of the specifics of ceramide function is still under investigation. Ceramides also serve as a precursor to more complex sphingolipids.[3]
Ceramides can only exist in membranes but they have a rapid flip rate between leaflets. This means they cannot leave the organelle they are created in, but will have access to binding proteins and enzymes on both sides of the bilayer. As such, ceramides can usually only be modified by enzymes that exist in the membrane compartment that the ceramide was produced in.[4]
Complex Sphingolipids
The synthesis of complex sphingolipids occurs in the Golgi apparatus.[3] The bulky headgroups of these sphingolipids make flipping between membrane leaflets very unlikely without the aid of flippases. This means that without flippases, sphingolipids are restricted to the lumenal Golgi leaflet/outer leaflet of the plasma membrane.[4] The two main type of complex sphingolipids are phospho- and glycol-sphingolipids.
Phosphosphingolipids
Phosphosphingolipids are linked by phosphodiester bonds.[5] In mammals, the major phosphosphingolipids are gomyelins (ceramide phosphocholines). In insects, it is ceramide phosphoethanolamines. The major phosphosphingolipids in fungi are phytoceramidesphosphoinositols and inositol phosphates. Aquatic organisms typically have sphingolipids where the phosphate has been replaced with a phosphono- or arsenate group.[2] Example phosphosphingolipids can be seen in Figure 1.5.3.
Glycosphingolipids
Glycosphingolipids have one or more carbohydrate groups in a glycosidic linkage (usually; ceramide phophonositols are an exception). The structure can be seen in Figure 1.5.4. There are four classifications of glycosphingolipids:
- Neutral glycosphingolipids: 1 or more uncharged sugars (i.e. glucose, galactose, N-acetylglucosamine, N-acetylgalacoseamine, fucose)[2]
- Acid glycosphingolipids: ionized functional groups (i.e. phosphate or sulfate) attached to neutral or charged sugars[2]
- Basic glycosphingolipids[5]
- Amphoteric glycosphingolipids[5]
Naming of Sphingolipids
Sphingolipids are named using IUPAC nomenclature for lipids (http://www.chem.qmul.ac.uk/iupac/lipid/). The most common sphingoid base is sphingosine. The official names of sphingosine are (2S,3R,4E)-2-aminooctadec-4-ene-1,3-diol or D-erythro-sphingosine & E-sphing-4-enine. The abbreviation is d18:1. The first number represents the number of carbon atoms. The second number is the number of double bonds. In this case, the letter ‘d’ refers to the 2 (di-) hydroxyl groups.[2,5]
Biological Functions
There are many biological functions associated with sphingolipids, including cellular regulation, signaling and metabolism.
Biophysical Properties
Sphingolipids tend to form clustered structured called microdomains, rafts, or caveolae (when containing caveolin). Saturated alkyl sidechains allow for strong van der Waals interactions. Ceramide hydroxyls, amide bonds, and polar headgroups allow for hydrogen bonding and dipole interactions. Sphingolipids are enriched in growth factor receptors, transporteres and proteins with glycosylphosphatidylinositiol-lipid anchors. Sphingolipids help stabilize the membrane bilayer which helps structures such as the lamellar bodies that maintain the permeability of skin. Their water solubility allows for rapid movement between membranes. Sphingolipids can exist in cationic and neutral forms.[2]
There are three major phases that a lipid membrane can take (Figure 1.5.5).
- In the gel phase, the lipid bilayers are in the solid state. The packing of the lipid molecules is very tight and ordered, leading to little movement of lipids across the membrane surface.
- In the liquid disordered phase, are highly fluid states in which the individual lipids can move unhindered across the surface of the membrane. The packing of the lipids is highly irregular and the lipids themselves are often kinked due to unsaturated fatty acids.
- The liquid ordered phase in a cross between the gel phase and the liquid disordered phase. There is still the relatively easy movement of lipid molecules across the membrane, but the membrane itself is more tightly packed, leading to a more solid structure. This phase comes about through the presence of sterol molecules within the membrane.
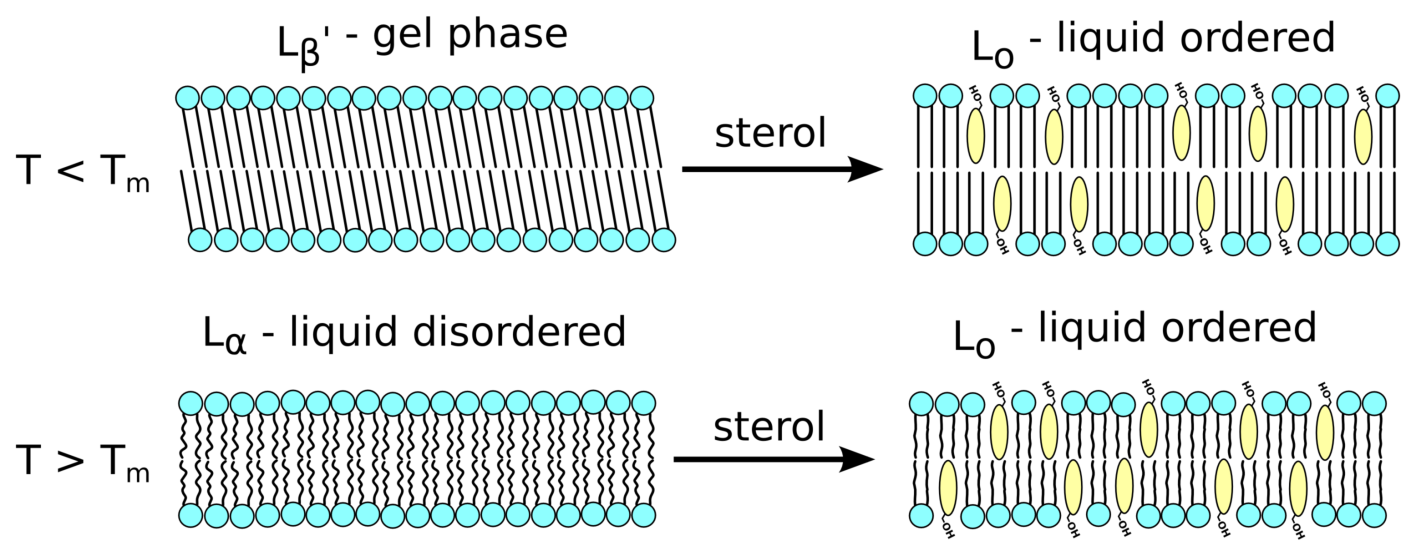
Membrane rafts are caused by a phase separation in the membrane. They occur when the liquid ordered phase and the disordered phase coexist. Tight packing of lipids is key to raft formation. In the liquid ordered phase, like the ordered gel phase, lipids are elongated and tightly packed, but they still have a higher degree of lateral mobility.[6] Figure 1.5.7 shows a membrane with a lipid raft.
Membrane rafts are abundant in membranes that are cholesterol and sphingolipid rich (i.e. the plasma membrane). They are important in sorting in the trans-Golgi network, sorting in the endocytic pathway, as a docking site for pathogens and toxins, and as integrin receptors.[6]

Cellular Regulation
Cellular sphingolipids are located in many places throughout the cell. They are found in the outer leaflet of the plasma membrane, in the lumen of intracellular vesicles/organelles (i.e. endosomes, Golgi membranes), and in undefined locations in the mitochondria, nuclei, and intracellular compartments. This abundance makes them ideal candidates for cell regulation.[2]
Cellular sphingolipids interact with complementary ligands (i.e. extracellular matrix proteins/receptors), with other carbohydrates (i.e. glycosynapse) and with proteins on the same cell surface. Through these interactions, they can control the location of a protein (i.e. in membrane rafts) and modify the conformation of the protein and its activity. They can also be recognized by viruses, bacteria and bacterial toxins as a means of attachment and entry.[2]
Ceramides have multiple roles in membrane biogenesis and regulation. They can influence ER to Golgi trafficking. They can help with the formation of autophagosomes. It is also likely that they influence the ER interactions with the nuclear membrane, outer membrane of mitochondria, and the plasma membrane.[2]
Cellular Signaling
Sphingolipids affect cell signaling by influencing the properties of receptors directly. The exact pathway for signal transduction varies depending on the exact function of the sphingolipids but there is a general pathway.[2]
- Receptors are activated by cytokines (i.e. IL1, tumor necrosis factor-ω), growth factors (i.e. platelet derived growth factor), or other agonists.
- Hydrolysis of membrane sphingolipid to ceramide is induced.
- Sphingolipids either serve as a lipid second messenger, or can be converted to downstream metabolites (i.e. sphingosine, sphingosine 1-phosphate, ceramide 1-phosphate).
- Downstream targets (i.e. proteins kinases, phosphoprotein phosphatases) that control cell behavior are either activated or inhibited.
Sometimes, multiple sphingolipids within the same cell have opposing signaling function. For example, ceraminde and sphingosine 1-phosphate signal for conflicting functions of cell growth. One signals for the inhibition of growth, while the other signals for the stimulation of growth. (Or in other words, the induction vs. inhibition of apoptosis.) The cellular pathway taken is determined by a ceraminde/sphingosine 1-phosophate “rheostat” in deciding between growth arrest/apoptosis and proliferation/survial.[2]
Because sphingolipids are complex molecules, they can take diverse input signals and produce multiple coordinated outputs. Signaling from sphingolipids also alters membrane structure and behavior of other membrane receptors and/or proteins.[2]
Metabolism
Sphingolipid base acylation is done through ceramide synthases which are highly specific for acyl chain lengths.[2] Regulation occurs through control of enzyme expression, post-translational modifications, and allosteric mechanisms. These can be cell specific – they can control sphingolipid synthesis during specific phases of the cell cycle, in response to specific signals.[3] Figure 1.5.8 shows several metabolic pathways of sphingolipids.
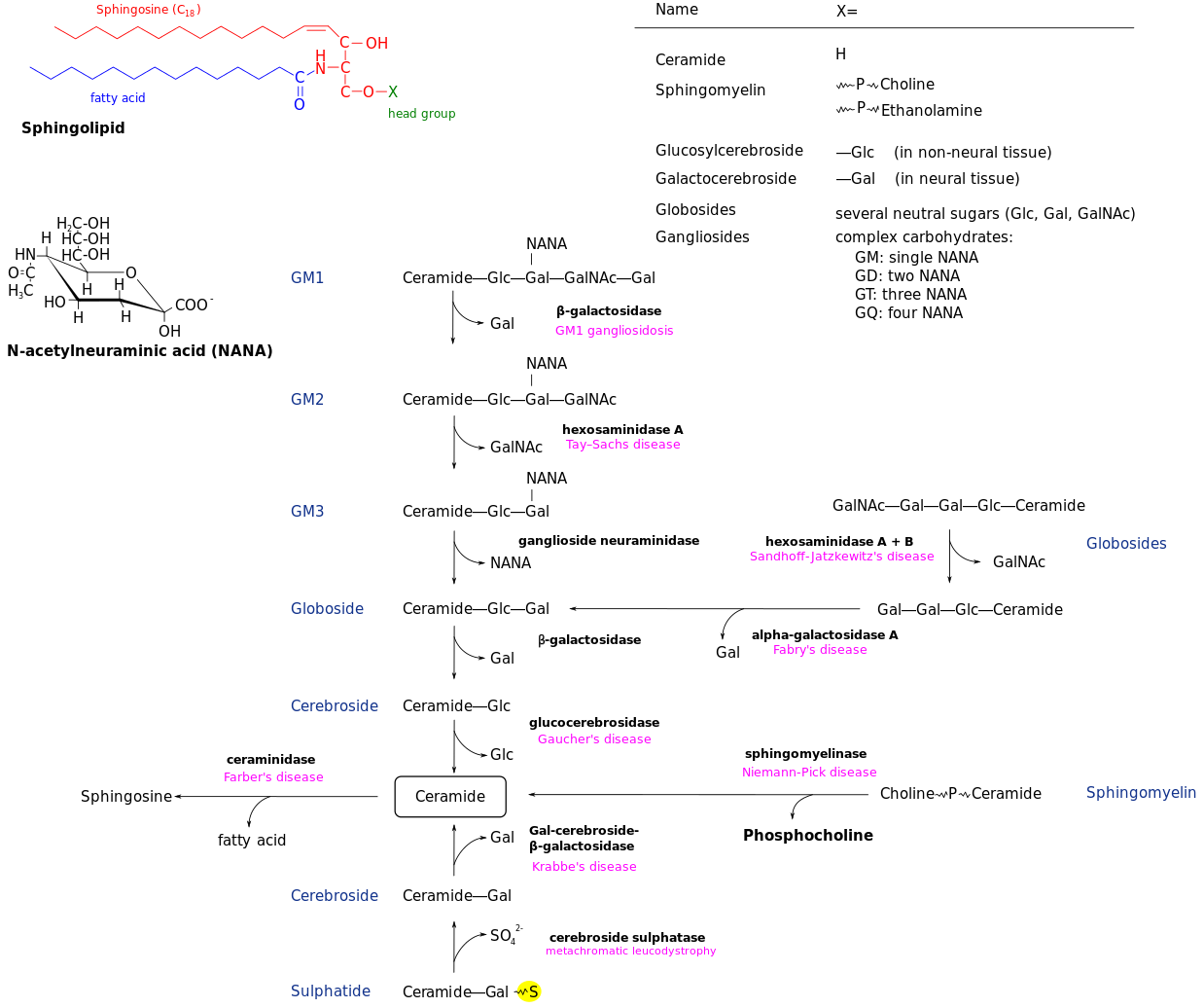
During sphingolipid metabolism, sphingomyelin turnover produces signaling metabolites and alters the structure of membrane domains, depending on its presence. In addition, changing the glycosphingolipid composition affects the properties of the cell surface and its interaction with the extracellular matrix and neighboring cells. Because of this, sphingolipid metabolism and signaling are basically the same.[2]
Sources
- Thudichum, J. L. W. 1884. “A Treatise on the Chemical Constitution of Brain”. Bailliere, Tindall, and Cox, London.
- Hirabayashi, Y., Igarashi, Y., Merrill, A.H. (2006). Sphingolipid Biology Springer, Japan.
- Futerman, A. H., Hannun, Y. A. (2004). "The complex life of simple sphingolipids." EMBO Rep 5(8): 777-782.
- Gault, C., et al. (2010). "An overview of sphingolipid metabolism: from synthesis to breakdown." Adv Exp Med Biol 688: 1-23.
- Fahy, E., et al. (2005). "A comprehensive classification system for lipids." J Lipid Res 46(5): 839-861.
- Brown, D. A. and E. London (2000). "Structure and Function of Sphingolipid- and Cholesterol-rich Membrane Rafts." J Biol. Chem. 275(23): 17221-17224.