5.6: Near-field Scanning Optical Microscopy (NSOM)
( \newcommand{\kernel}{\mathrm{null}\,}\)
Near-field scanning optical microscopy (NSOM), also called scanning near-field optical microscopy (SNOM), is a scanning probe technique that overcomes the diffraction barrier in traditional far-field optical microscopy. Conventional optical microscopy techniques are limited by the diffraction of light and the resolution is limited to roughly 250 nm, which make it very difficult to resolve the domains or clusters in the cellular membranes[1]. The basic idea in NSOM is to confine the illuminating light to nanometric dimensions to break the diffraction limit that cannot be achieved by traditional far-field optical microscopy[2]. Thus, NSOM can produce high-resolution topographical and optical images to study biological membranes. Figure 5.6.1 shows the diffraction of light and the near-field in the NSOM fiber probe.

Near-field Theory
In traditional far-field optical microscopy, the illumination source is a monochromatic plane wave[3]. The lens collecting the scattering light is placed several wavelengths of the illumination light far away from the sample surface. This causes the commonly known diffraction limit, that is far-field optical techniques are limited to resolve features approximately on the order of half of the wavelength of the illuminating light due to the diffraction of light. However, the classical NSOM uses a tapered optical fiber probe and an aperture that is much smaller than the wavelength of the light. As shown in Figure 5.6.2, after light passes through the cantilever tip with nano-aparture, a optical near-field (or evanescent field) on the far side of this tip can be created that is not diffraction limited[4]. Then the resolution in near-field microscopy is directly affected by the size of the aperture and independent of the wavelength of the light.

NSOM Instrumentation
Standard NSOM normally contains three parts, the illumination unit, the collection and redistribution unit and the detection module[3]. The illuminating light comes from the probe, goes through the aperture at the tip and interacts with the sample surface. As light passes through the sample, the absorption or the fluorescence produced by the labeled molecules on the sample surface can be collected. During the scan, the probe can be brought to the sample at a very small distance(<10 nm)[5]. The topographic and optical images showing the high spatial resolution can be generated simultaneously. The lateral resolution can be reached down to tens of nanometers, which is determined by the size of the aperture and sample-probe distance[6]. The probe tip movement is monitored and controlled by the feedback system and the x-y-z scanner (usually piezoelectric) to keep the tip within the near-field.
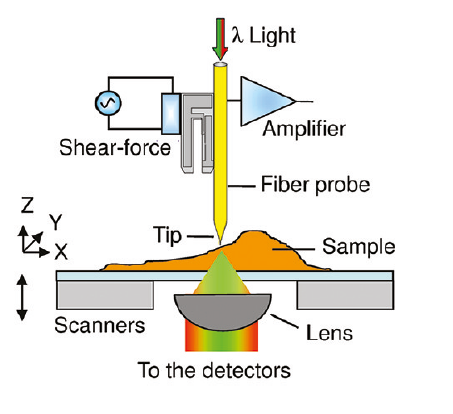
Figure 5.6.4 shows three common modes of NSOM measurements, which are illumination mode, collection mode and scattering mode for apertureless NSOM. In illumination mode, the evanescent field can be generated at the tip end when the illuminated light passes through the probe near the sample. Then the scattered light from the probe-sample system is collected to generate the NSOM image. In collection mode, the evanescent wave near sample is acquired by the local probe within near-field of sample. The dashed line means the critical angle. Besides aperture NSOM, the NSOM with apertureless probe was also developed, which use STM-like tip or AFM cantilever tip. The probe tip is also brought very close to the sample, and the scattering light is induced near sample.
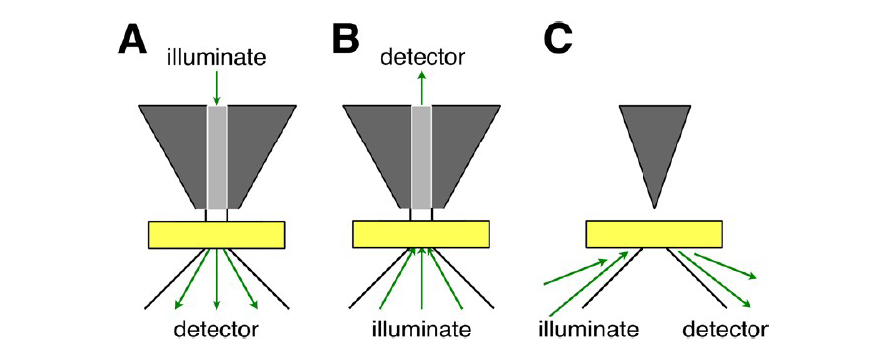
NSOM Fluorescence Imaging of Membranes
The combination of NSOM and the simultaneous fluorescence has been used to investigate the membrane systems such as lipid bilayers, lipid rafts, membrane receptor, clustering and other small domains on the membrane[2]. The near-field technique makes it possible to detect the small domains with high resolution that cannot be achieved by the traditional optical microscopes. Further, the specific assignments of detected domains can be made by comparing the simultaneous fluorescence mapping and the surface topography. Thus, the high-resolution images of NSOM combined with fluorescence can provide abundant information such as the size and specific features of different domains on the membrane, which is very helpful for us to understand the membrane organization and important function.
Supported lipid bilayers (SLB) studied by NSOM
Supported lipid bilayers (SLB) are phospholipid bilayers that contain different lipids, cholesterol or proteins. SLBs are significant model membranes that have been studied for a long time. NSOM help researchers to better understand the crucial factors in the small domains and structures in SLBs with high-resolution. NSOM images of SLBs can identify different domains of dipalmitoylphosphatidylcholine (DPPC), dilauroylphosphatidylcholine (DLPC) on membranes[5].
Lipid rafts studied by NSOM
For the heterogeneous lipid domains in cell membranes, also called lipid rafts, NSOM has been used to visualize the nanolandscape of ganglioside GM1 after tightening by its ligand cholera toxin (CTxB). Figure 5.6.4 demonstrates the resolution of NSOM being able to resolve nanodomains that were not possible with the conventional optical microscopes. NSOM was able to show the nanodomains were smaller than 120 nm.

Membrane protein receptors studied by NSOM
The formation of receptors nanoclusters on membrane could also be investigated by NSOM. For example, the distribution of receptor DC-SIGN, a transmembrane protein in immature dendritic cells (DC) has been studied by NSOM. As shown in Figure1.5, about 80% of the immature dendritic cells' protein receptors are approximately 185 nm in diameter. The "finger" domains are the main topographic feature of dendritic cells, where the colorful fluorescent areas indicate nanocluster distributions of DC-SIGN receptors.
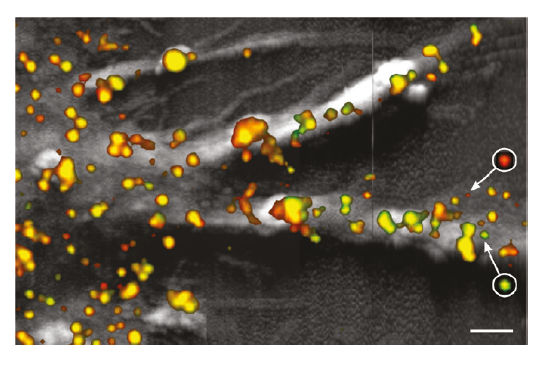
Summary
NSOM has great advantages in studying biological membranes over conventional optical microscopy. The combination of near-field technique and scanning probe techniques utilized by NSOM can generate high spatial resolution images beyond the diffraction limit, which is not possible in conventional optical microscopy. Combined with fluorescence technique, NSOM can show both topographic image and the simultaneous optical fluorescence image that can detect the small domains and the specific features in membrane systems.
References
- Sezgin, E., Super-resolution Optical Microscopy for Studying Membrane Structure and Dynamics. J. Phys.: Condens. Matter 2017, 29, 27
- Huckabay, H. A.; Armendariz, K. P.; Newhart, W. H.; Wildgen, S. M.; Dunn, R. C., Near-Field Scanning Optical Microscopy for High-Resolution Membrane Studies. Methods in molecular biology (Clifton, N.J.) 2013, 950, 373-394.
- Hecht, B.; Sick, B.; Wild, U. P.; Deckert, V.; Zenobi, R.; Martin, O. J. F.; Pohl, D. W., Scanning near-field optical microscopy with aperture probes: Fundamentals and applications. The Journal of Chemical Physics 2000, 112 (18), 7761-7774.
- Germany, WITec Wissenschaftliche Instrumente und Technologie GmbH, Ulm. https://www.witec.de/techniques/snom/ . Retrieved 2017-04-06.
- Raigoza, A. F.; Dugger, J. W.; Webb, L. J., Review: Recent Advances and Current Challenges in Scanning Probe Microscopy of Biomolecular Surfaces and Interfaces. ACS Applied Materials & Interfaces 2013, 5 (19), 9249-9261.
- Hinterdorfer, P.; Garcia-Parajo, M. F.; Dufrêne, Y. F., Single-Molecule Imaging of Cell Surfaces Using Near-Field Nanoscopy. Accounts of Chemical Research 2012, 45 (3), 327-3Near-Field Nanoscopy