1.6: Sterols and Sterol Induced Phases
- Page ID
- 870
\( \newcommand{\vecs}[1]{\overset { \scriptstyle \rightharpoonup} {\mathbf{#1}} } \)
\( \newcommand{\vecd}[1]{\overset{-\!-\!\rightharpoonup}{\vphantom{a}\smash {#1}}} \)
\( \newcommand{\id}{\mathrm{id}}\) \( \newcommand{\Span}{\mathrm{span}}\)
( \newcommand{\kernel}{\mathrm{null}\,}\) \( \newcommand{\range}{\mathrm{range}\,}\)
\( \newcommand{\RealPart}{\mathrm{Re}}\) \( \newcommand{\ImaginaryPart}{\mathrm{Im}}\)
\( \newcommand{\Argument}{\mathrm{Arg}}\) \( \newcommand{\norm}[1]{\| #1 \|}\)
\( \newcommand{\inner}[2]{\langle #1, #2 \rangle}\)
\( \newcommand{\Span}{\mathrm{span}}\)
\( \newcommand{\id}{\mathrm{id}}\)
\( \newcommand{\Span}{\mathrm{span}}\)
\( \newcommand{\kernel}{\mathrm{null}\,}\)
\( \newcommand{\range}{\mathrm{range}\,}\)
\( \newcommand{\RealPart}{\mathrm{Re}}\)
\( \newcommand{\ImaginaryPart}{\mathrm{Im}}\)
\( \newcommand{\Argument}{\mathrm{Arg}}\)
\( \newcommand{\norm}[1]{\| #1 \|}\)
\( \newcommand{\inner}[2]{\langle #1, #2 \rangle}\)
\( \newcommand{\Span}{\mathrm{span}}\) \( \newcommand{\AA}{\unicode[.8,0]{x212B}}\)
\( \newcommand{\vectorA}[1]{\vec{#1}} % arrow\)
\( \newcommand{\vectorAt}[1]{\vec{\text{#1}}} % arrow\)
\( \newcommand{\vectorB}[1]{\overset { \scriptstyle \rightharpoonup} {\mathbf{#1}} } \)
\( \newcommand{\vectorC}[1]{\textbf{#1}} \)
\( \newcommand{\vectorD}[1]{\overrightarrow{#1}} \)
\( \newcommand{\vectorDt}[1]{\overrightarrow{\text{#1}}} \)
\( \newcommand{\vectE}[1]{\overset{-\!-\!\rightharpoonup}{\vphantom{a}\smash{\mathbf {#1}}}} \)
\( \newcommand{\vecs}[1]{\overset { \scriptstyle \rightharpoonup} {\mathbf{#1}} } \)
\( \newcommand{\vecd}[1]{\overset{-\!-\!\rightharpoonup}{\vphantom{a}\smash {#1}}} \)
\(\newcommand{\avec}{\mathbf a}\) \(\newcommand{\bvec}{\mathbf b}\) \(\newcommand{\cvec}{\mathbf c}\) \(\newcommand{\dvec}{\mathbf d}\) \(\newcommand{\dtil}{\widetilde{\mathbf d}}\) \(\newcommand{\evec}{\mathbf e}\) \(\newcommand{\fvec}{\mathbf f}\) \(\newcommand{\nvec}{\mathbf n}\) \(\newcommand{\pvec}{\mathbf p}\) \(\newcommand{\qvec}{\mathbf q}\) \(\newcommand{\svec}{\mathbf s}\) \(\newcommand{\tvec}{\mathbf t}\) \(\newcommand{\uvec}{\mathbf u}\) \(\newcommand{\vvec}{\mathbf v}\) \(\newcommand{\wvec}{\mathbf w}\) \(\newcommand{\xvec}{\mathbf x}\) \(\newcommand{\yvec}{\mathbf y}\) \(\newcommand{\zvec}{\mathbf z}\) \(\newcommand{\rvec}{\mathbf r}\) \(\newcommand{\mvec}{\mathbf m}\) \(\newcommand{\zerovec}{\mathbf 0}\) \(\newcommand{\onevec}{\mathbf 1}\) \(\newcommand{\real}{\mathbb R}\) \(\newcommand{\twovec}[2]{\left[\begin{array}{r}#1 \\ #2 \end{array}\right]}\) \(\newcommand{\ctwovec}[2]{\left[\begin{array}{c}#1 \\ #2 \end{array}\right]}\) \(\newcommand{\threevec}[3]{\left[\begin{array}{r}#1 \\ #2 \\ #3 \end{array}\right]}\) \(\newcommand{\cthreevec}[3]{\left[\begin{array}{c}#1 \\ #2 \\ #3 \end{array}\right]}\) \(\newcommand{\fourvec}[4]{\left[\begin{array}{r}#1 \\ #2 \\ #3 \\ #4 \end{array}\right]}\) \(\newcommand{\cfourvec}[4]{\left[\begin{array}{c}#1 \\ #2 \\ #3 \\ #4 \end{array}\right]}\) \(\newcommand{\fivevec}[5]{\left[\begin{array}{r}#1 \\ #2 \\ #3 \\ #4 \\ #5 \\ \end{array}\right]}\) \(\newcommand{\cfivevec}[5]{\left[\begin{array}{c}#1 \\ #2 \\ #3 \\ #4 \\ #5 \\ \end{array}\right]}\) \(\newcommand{\mattwo}[4]{\left[\begin{array}{rr}#1 \amp #2 \\ #3 \amp #4 \\ \end{array}\right]}\) \(\newcommand{\laspan}[1]{\text{Span}\{#1\}}\) \(\newcommand{\bcal}{\cal B}\) \(\newcommand{\ccal}{\cal C}\) \(\newcommand{\scal}{\cal S}\) \(\newcommand{\wcal}{\cal W}\) \(\newcommand{\ecal}{\cal E}\) \(\newcommand{\coords}[2]{\left\{#1\right\}_{#2}}\) \(\newcommand{\gray}[1]{\color{gray}{#1}}\) \(\newcommand{\lgray}[1]{\color{lightgray}{#1}}\) \(\newcommand{\rank}{\operatorname{rank}}\) \(\newcommand{\row}{\text{Row}}\) \(\newcommand{\col}{\text{Col}}\) \(\renewcommand{\row}{\text{Row}}\) \(\newcommand{\nul}{\text{Nul}}\) \(\newcommand{\var}{\text{Var}}\) \(\newcommand{\corr}{\text{corr}}\) \(\newcommand{\len}[1]{\left|#1\right|}\) \(\newcommand{\bbar}{\overline{\bvec}}\) \(\newcommand{\bhat}{\widehat{\bvec}}\) \(\newcommand{\bperp}{\bvec^\perp}\) \(\newcommand{\xhat}{\widehat{\xvec}}\) \(\newcommand{\vhat}{\widehat{\vvec}}\) \(\newcommand{\uhat}{\widehat{\uvec}}\) \(\newcommand{\what}{\widehat{\wvec}}\) \(\newcommand{\Sighat}{\widehat{\Sigma}}\) \(\newcommand{\lt}{<}\) \(\newcommand{\gt}{>}\) \(\newcommand{\amp}{&}\) \(\definecolor{fillinmathshade}{gray}{0.9}\)Sterols are a class of hydrophobic ringed lipid molecules found in biological membranes throughout eukarya. Sterols can comprise greater than 50% of the membrane lipid content in cells, and are known to alter membrane fluidity and structure [1]. Though the mechanisms of these effects are still under debate, sterols have been shown to induce altered lipid phases in bilayer systems, generally increasing the order of acyl chains while maintaining lipid translational fluidity. Their actions are thought to be essential to the formation of lipid rafts with various effects on cell biology and serve as precursors to steroid hormones in animals.
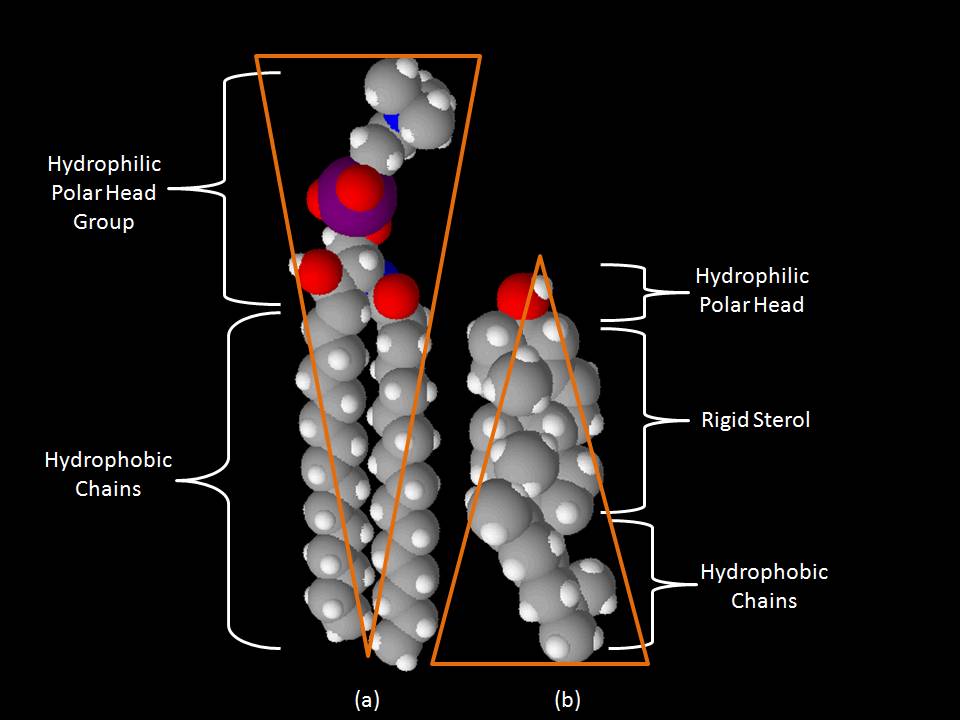
Sterol Structure
All naturally occurring sterols are comprised of a hydrocarbon tetrameric fused ring structure and acquire a small degree of polarity from a 3-hydroxyl in the β configuration (fig 2); α-hydroxyl sterols are not found in natural membranes. The most common sterols also have a short aliphatic tail on the opposite end on C17. In lipid bilayers the hydroxyl acts as the polar head group and orients towards the phospholipid head groups of the bilayer, while the rings and short hydrophobic tail extend into the hydrophobic core of the membrane, aligning with the acyl chains of the phospholipids. The topography of sterols is such that the α side of the relatively rigid ring structure is flat while the β side has protruding methyl groups; this structure constrains their interactions with lipids allowing significant contact on the flat surface.
Common Sterols
Many different sterols are found in biological membranes and are specific to certain phyla. Subtle differences in their structure can change their effect on membrane structure and dynamics as discussed below. Cholesterol is the most common sterol in animal membranes accounting for approximately 20-30% of sterols found therein [2]. It is characterized by a single double bond in ring B (C5-C6) and the lack of a branching carbon at position 24. Ergosterol is found in the membranes of fungi and protists and differs from cholesterol by containing two more carbon-carbon double bonds (one in ring B, another between carbons 22 and 23 in the tail region) and a methyl group at position 24.
Sterols from plants are known as phytosterols. Common phytosterols include campesterol, stigmasterol and sitosterol, though hundreds of different compounds have been isolated from plants. Hopanoids are pentacyclic compounds that share a similar structure with sterols and are often found in prokaryotic membranes, where they are thought to have an effect on membrane stability and structure similar to sterols [3].
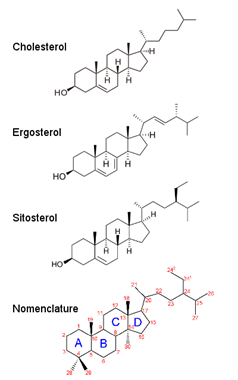
Liquid-ordered Phase (lo)
Sterols have pronounced effects on the phase behavior of lipid membranes. When added to lipids in the gel phase (Lβ') sterols tend to liquefy the membrane structure by disrupting the highly ordered tilted structure of the hydrocarbon chains. At low temperatures this induces the liquid crystal phase (Lc') where the lipid tails are fully extended, but not tilted [4]. Conversely, cholesterol tends to solidify lipids in the liquid disordered phase (Lα). In both cases, higher cholesterol concentration leads to the formation of an intermediate phase, known as the liquid ordered (lo) phase. In the lo phase, the phospholipid tails have higher order similar to the gel phase, but maintain lateral fluidity similar to the Lα phase [2,5] (fig. 3). The area per molecule is also reduced (see condensation effect below)

Lipid phase diagrams (fig 4) show the phase coexistence of lo and either Lα or the ripple phase Pβ (sometimes called pre-transition phase), depending on temperature in lower cholesterol content. As the relative concentration of sterol in the membrane increases, the lo phase dominates as all phospholipids come in near contact with sterols [7]. The ordering of saturated acyl chains in the presence of cholesterol has been studied by many different methods including 2H-NMR, EPR, and MD simulations [2], which show that sterols induce order in neighboring fatty acid tails. Headgroup type, tail length and saturation, and whether the lipids are glycerol or sphingosine-based all influence the effects of sterols [2]. Notably, sterols have the most pronounced effect on the phase behavior of long saturated phospholipids like dipalmitoylphosphatidylcholine (DPPC); however, the presence of one saturated tail is sufficient for the effect [7]. Likewise, sterols with an α conformation or methyl groups in the trans conformation are incapable of affecting the phase behavior of lipids [1].
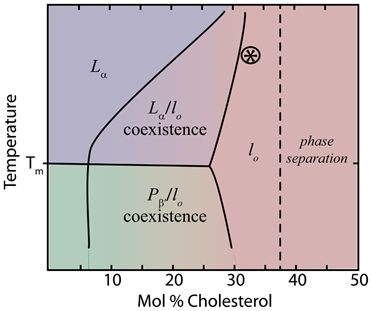
Cholesterol and the main phase transition
The effect of cholesterol on lipid phases has been extensively studied with a method known as differential scanning calorimetry (DSC). With DSC the rate of heat flow can be measured as the temperature of a sample is increased or decreased at a constant rate. When the sample transitions between phases, the excess heat flow is recorded as a spike around the temperature at which that phase transition occurs. When heated or cooled past their phase transitions, pure lipids will display a sharp peak. The addition of sterols leads to a dampening and broadening of the enthalpy spikes associated with pre-transition and the main phase transition, effectively eliminating the phase transitions at higher cholesterol concentrations as the membrane enters the lo phase [1]. This effect is dependent on the type and amount of sterol as well as the type of phospholipid [9]. For example, a precursor to cholesterol known as lathosterol differs in structure only by the placement of one double bond. This small difference means the lathosterol decreases the temperature of the main phase transition while cholesterol increases it, though cholesterol is eliminates the transition at high molar percent while lathosterol does not [9]
The Condensation Effect
When cholesterol is added to a membrane, the 2-dimensional area per molecule decreases while the membrane thickness increases; this is known as the condensation effect. The mechanism for this effect is unclear [4], but the rigid structure of cholesterol means that the reduction in surface area must be due to changes in the order of the lipid tails (fig 5). In DMPC membranes, increasing cholesterol from 0 to 50 mol% can lead to 25% reduction in area per DMPC molecules [2]. This condensation augments membrane strength and decreases permeability, and is strongest when paired with phosphatidylcholine-based lipids [10].

Sterols in Rafts
In biological membranes sterols are essential components of lipid rafts. Cholesterol makes a hydrogen bond with the sphingosine head group and preferentially associates with the fatty acid tails of sphingomyelin over other lipid tails. Cholesterol and sphingomyelin together solidify the membrane and decrease the lateral diffusion of lipids leading to long-lasting lo domains amid Lαlipids [11]. The lipid characteristics of rafts are thought to be important in determining membrane protein function.
Steroid Hormones
In animals, cholesterol serves as a precursor for all steroid hormones such as estradiol, aldosterone and cortisol, which act via intracellular receptors to regulate gene transcription in target tissues. These hormones are vital to nearly all physiological function including growth and reproduction, the stress response, water balance and metamorphosis (in invertebrates).
References
- R.A. Demel, B. De Kruyff, Biochimica et Biophysica Acta (BBA) - Reviews on Biomembranes 457 (1976) 109.
- T. Róg, M. Pasenkiewicz-Gierula, I. Vattulainen, M. Karttunen, Biochimica et Biophysica Acta (BBA) - Biomembranes 1788 (2009) 97.
- M. Rohmer, P. Bouvier-Nave, G. Ourisson, Journal of General Microbiology 130 (1984) 1137.
- F. de Meyer, B. Smit, Proceedings of the National Academy of Sciences 106 (2009) 3654.
- K.J. Tierney, D.E. Block, M.L. Longo, Biophys J 89 (2005) 2481.
- R. Faller. BPH 241 Lecture slides, UC Davis (2014)
- C.L. Armstrong, D. Marquardt, H. Dies, N. Kučerka, Z. Yamani, T.A. Harroun, J. Katsaras, A.-C. Shi, M.C. Rheinstädter, PLoS ONE 8 (2013) e66162.
- M.G.K. Benesch, R.N. McElhaney, Biochimica et Biophysica Acta (BBA) - Biomembranes 1838 (2014) 1941.
- M.G. Benesch, D.A. Mannock, R.N. Lewis, R.N. McElhaney, Biochemistry 50 (2011) 9982.
- P.J. Quinn and C. Wolfe, Biochimica et Biophysica Acta 1788 (2009) 33
- P.S. Niemelä, S. Ollila, M.T. Hyvönen, M. Karttunen, I. Vattulainen, PLoS Comput Biol 3 (2007) e34.
Contributors and Attributions
- Ian Kimball