12.2: Blackbody Radiation
( \newcommand{\kernel}{\mathrm{null}\,}\)
Learning Objectives
- Explain Max Planck’s contribution to the development of quantum mechanics, explaining blackbody radiation.
- Explain the significance of Planck's constant and the smallness of its value.
Ultraviolet Catastrophe
The history of quantum mechanics starts with the study of thermal physics. With the development of theory of electromagnetism and thermodynamics in the 18th century, physicists felt confident enough to attempt to develop a theory of blackbody radiation, which describes the EM spectrum radiated by a hot solid. It is called blackbody radiation, because an ideal radiator is jet black. It absorbs all radiation incident on it, and thus it can be at thermal equilibrium with the surrounding while emitting more radiation than a white object, which reflects radiation. So such a hypothetical object is called a blackbody. The first, classical attempt at theoretical description of this phenomenon was nothing short of a catastrophe. Experimentally observed spectrum showed less intensity of EM radiation at both the very short wavelength limit and the very long wavelength limit, but the classical theory prediction predicted ever-increasing intensity at shorter and shorter wavelengths. (See Figure 12.2.1.)
This result came to be called ultraviolet catastrophe, due to the catastrophically large intensity of radiation predicted in the ultraviolet range of EM spectrum. This problem stumped many physicists until Max Planck came up with a novel suggestion.
Planck Law
The spectrum of blackbody radiation was extensively studied and well known. The German physicist Max Planck (1858–1947) first guessed a functional form of intensity dependence on the wavelength of EM radiation, which is now known as the Planck Law. The Planck Law was a good fit to the experimental results (shown in Figure 12.2.2), tapering off both at the long-wavelength and the short-wavelength limit, but it was only a phenomenological description that did not include a satisfying explanation of why it was so.
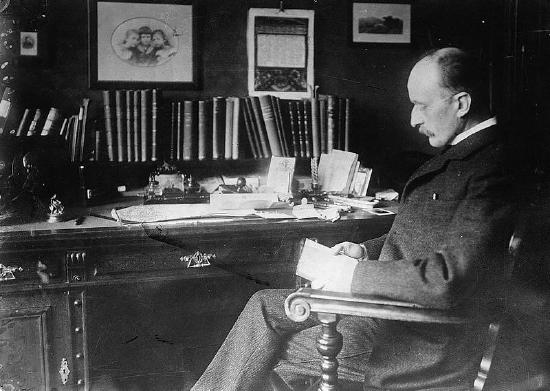
This led Planck to look for a reason why intensity of radiation should decrease at the short-wavelength, or high-frequency limit. Using the idea that atoms and molecules in a body act like oscillators to absorb and emit radiation, he guessed that the energy of these oscillators are quantized. That is, the energy of the oscillators could change only by a discrete amount, and this discrete amount of energy change was ΔE=hf, where f is frequency of the oscillator and h is a fundamental constant of nature that we now call Planck's constant, given by
h=6.626×10−34 J⋅s.
Without getting into the detailed mathematical derivation, we can see intuitively why this assumption would lead to the result that Planck was looking for. An object at thermal equilibrium has a certain amount of thermal energy, which is associated with kinetic energy of microscopic movements and vibrations. If this energy is randomly distributed, both very high-frequency oscillators and very low-frequency oscillators would have the same thermal energy on average. But if it was true that this thermal energy is quantized, and it has to come in integer units of hf, then at very high frequencies, there is not going to be enough thermal energy for even one unit of hf. This places an upper limit on maximum possible frequency of these oscillators, for a given amount of thermal energy per oscillator, resulting in the tapering behavior for intensity of radiation emitted by these oscillators at the higher frequency (or shorter wavelengths).
This model would also explain why the peak wavelength shifts to the shorter wavelength (higher frequency) at higher temperatures. At higher temperatures, there is more thermal energy available per oscillator, so the upper limit on maximum possible frequency will be higher, since larger unit size hf is allowed.
Note that Planck’s constant h is a very small number. So for an infrared frequency of 1014 Hz being emitted by a blackbody, for example, the difference between energy levels is only ΔE=hf=(6.63×10−34 J⋅s)(1014 Hz)=6.63×10−20 J or about 0.4 eV. This 0.4 eV of energy is significant compared with typical atomic energies, which are on the order of an electron volt, or thermal energies, which are typically fractions of an electron volt. But on a macroscopic or classical scale, energies are typically on the order of joules. Even though the macroscopic energies are quantized, the quantum steps are too small to be noticed. This is an example of the correspondence principle. For a large object, quantum mechanics produces results indistinguishable from those of classical physics.
Section Summary
- The first indication that energy is sometimes quantized came from blackbody radiation, which is the emission of EM radiation by an object that absorbs all radiation incident on it.
- The failure of classical theory to successfully explain blackbody radiation is called "ultraviolet catastrophe," after the large intensity of short-wavelength radiation incorrectly predicted.
- Planck derived the Planck Law by assuming that energy of oscillators is quantized and can change only by amount ΔE=hf, where h, Planck's constant is,
h=6.626×10−34 J⋅s.
Glossary
- blackbody
- an ideal radiator, which emits thermal radiation ideally and absorbs all radiation incident on it at all wavelengths
- blackbody radiation
- a thermal radiation, usually modeled for a blackbody
- ultraviolet catastrophe
- failure of classical theory to correctly describe blackbody radiation at short wavelengths
- Planck’s constant
- h=6.626×10−34 J⋅s
- Planck law
- first successful theoretical description of thermal radiation which used quantization of energy hypothesis