23.7: Small Oscillations
( \newcommand{\kernel}{\mathrm{null}\,}\)
Any object moving subject to a force associated with a potential energy function that is quadratic will undergo simple harmonic motion,
U(x)=U0+12k(x−xeq)2
where k is a “spring constant”, xeq is the equilibrium position, and the constant U0 just depends on the choice of reference point xref for zero potential energy, U(xref)=0,
0=U(xref)=U0+12k(xref−xeq)2
Therefore the constant is
U0=−12k(xref−xeq)2
The minimum of the potential x0 corresponds to the point where the x -component of the force is zero,
dUdx|x=x0=2k(x0−xeq)=0⇒x0=xeq
corresponding to the equilibrium position. Therefore the constant is U(x0)=U0 and we rewrite our potential function as
U(x)=U(x0)+12k(x−x0)2
Now suppose that a potential energy function is not quadratic but still has a minimum at x0. For example, consider the potential energy function
U(x)=−U1((xx1)3−(xx1)2)
(Figure 23.22), which has a stable minimum at x0,
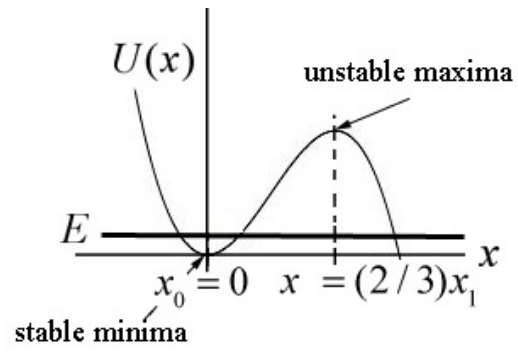
When the energy of the system is very close to the value of the potential energy at the minimum U(x0), we shall show that the system will undergo small oscillations about the minimum value x0. We shall use the Taylor formula to approximate the potential function as a polynomial. We shall show that near the minimum x0 we can approximate the potential function by a quadratic function similar to Equation (23.7.5) and show that the system undergoes simple harmonic motion for small oscillations about the minimum x0.
We begin by expanding the potential energy function about the minimum point using the Taylor formula
U(x)=U(x0)+dUdx|x=x0(x−x0)+12!d2Udx2|x=x0(x−x0)2+13!d3Udx3|x=x0(x−x0)3+⋯
where 13!d3Udx3|x=x0(x−x0)3 is a third order term in that it is proportional to (x−x0)3, and d3Udx3|x=x0,d2Udx2|x=x0, and dUdx|x=x0 are constants. If x0 is the minimum of the potential energy, then the linear term is zero, because
dUdx|x=x0=0
and so Equation ((23.7.7)) becomes
U(x)≃U(x0)+12d2Udx2|x=x0(x−x0)2+13!d3Udx3|x=x0(x−x0)3+⋯
For small displacements from the equilibrium point such that |x−x0| is sufficiently small, the third order term and higher order terms are very small and can be ignored. Then the potential energy function is approximately a quadratic function,
U(x)≃U(x0)+12d2Udx2|x=x0(x−x0)2=U(x0)+12keff(x−x0)2
where we define keff, the effective spring constant, by
keff≡d2Udx2|x=x0
Because the potential energy function is now approximated by a quadratic function, the system will undergo simple harmonic motion for small displacements from the minimum with a force given by
Fx=−dUdx=−keff(x−x0)
At x=x0, the force is zero
Fx(x0)=dUdx(x0)=0
We can determine the period of oscillation by substituting Equation (23.7.12) into Newton’s Second Law
−keff(x−x0)=meffd2xdt2
where meff is the effective mass. For a two-particle system, the effective mass is the reduced mass of the system.
meff=m1m2m1+m2≡μred
Equation (23.7.14) has the same form as the spring-object ideal oscillator. Therefore the angular frequency of small oscillations is given by
ω0=√keffmeff=√(d2Udx2|x=x0)/meff
Example 23.6: Quartic Potential
A system with effective mass m has a potential energy given by
U(x)=U0(−2(xx0)2+(xx0)4)
where U0 and x0 are positive constants and U(0)=0 (a) Find the points where the force on the particle is zero. Classify these points as stable or unstable. Calculate the value of U(x)/U0 at these equilibrium points. (b) If the particle is given a small displacement from an equilibrium point, find the angular frequency of small oscillation.
Solution: (a) A plot of U(x)/U0 as a function of x/x0 is shown in Figure 23.23.
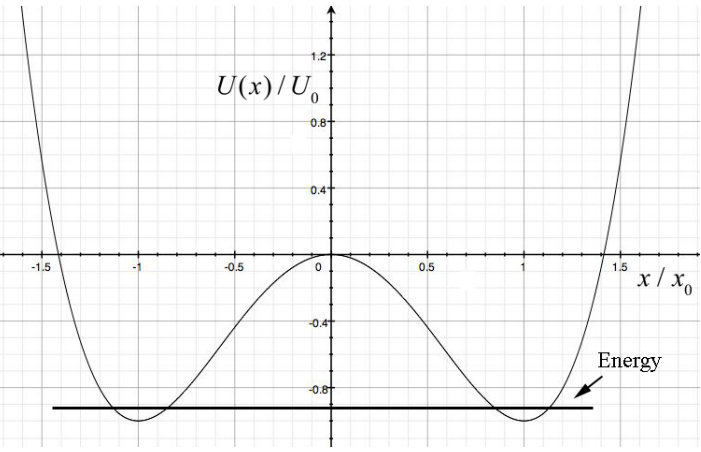
The force on the particle is zero at the minimum of the potential energy,
0=dUdx=U0(−4(1x0)2x+4(1x0)4x3)=−4U0x(1x0)2(1−(xx0)2)⇒x2=x20 and x=0
The equilibrium points are at x=±x0 which are stable and x = 0 which is unstable. The second derivative of the potential energy is given by
d2Udx2=U0(−4(1x0)2+12(1x0)4x2)
If the particle is given a small displacement from x=x0 then
d2Udx2|x=x0=U0(−4(1x0)2+12(1x0)4x20)=U08x20
(b) The angular frequency of small oscillations is given by
ω0=√d2Udx2|x=x0/m=√8U0mx20
Example 23.7: Lennard-Jones 6-12 Potential
A commonly used potential energy function to describe the interaction between two atoms is the Lennard-Jones 6-12 potential
U(r)=U0[(r0/r)12−2(r0/r)6];r>0
where r is the distance between the atoms. Find the angular frequency of small oscillations about the stable equilibrium position for two identical atoms bound to each other by the LennardJones interaction. Let m denote the effective mass of the system of two atoms.
Solution: The equilibrium points are found by setting the first derivative of the potential energy equal to zero,
0=dUdr=U0[−12r120r−13+12r60r−7]=U012r60r−7[−(r0r)6+1]
The equilibrium point occurs when r=r0 The second derivative of the potential energy function is
d2Udr2=U0[+(12)(13)r120r−14−(12)(7)r60r−8]
Evaluating this at r=r0 yields
d2Udr2|r=r0=72U0r−20
The angular frequency of small oscillation is therefore
ω0=√d2Udr2|r=r0/m
=√72U0/mr20